Available online: 09/04/2019
Editorial
REC Interv Cardiol. 2020;2:310-312
The future of interventional cardiology
El futuro de la cardiología intervencionista
Emory University School of Medicine, Atlanta, Georgia, United States
The arrival of drug-eluting stents (DES) nearly 2 decades ago ignited a revolution in the field of interventional cardiology. The addition of antiproliferative drugs to the stent platform reduced the rate of in-stent restenosis significantly and increased the number of patients that, to this date, have benefited from percutaneous revascularization.1,2 However, its Achilles tendon was initially the higher rate of late stent thrombosis involved. The permanent polymer used in the first generation of DES induced inflammatory and hypersensitivity reactions that delayed endothelization. A health alert followed3 that made the medical community recommend extended courses of dual antiplatelet therapy and put into question the convenience of DES. Beyond the increased rate of stent thrombosis, the first-generation of this type of stents had additional limitations: they were stainless-steel platforms with up to 140 µm thick struts, worse stent navigability, and fewer crossing capabilities. Also, the stent maximum expansion was limited (3.5 mm in the Cypher stent, Cordis Corp.), which occasionally prevented treating left main coronary artery lesions. With the passing of time, this stent technology has been refined to a point that there has been a major overhaul in its 3 main components: platform, polymer, and antiproliferative drug.
The changes made to the stent platform have been the development of more biocompatible alloys that have reduced strut thickness significantly and, consequently, improved stent navigability. Additionally, radial strength has been preserved to prevent recoil (table 1). Most of the stents commonly used today consist of cobalt-chromium or platinum-chromium alloys. The open-cell design has won the battle over the closed-cell design because it reduces the number of inter-strut links, the degree of jailed side-branch while improving stent navigability and conformability. Also, the new generation of DES have wider overexpansion limits (4.5 mm to 6 mm), which allows us to treat left main coronary artery lesions by adapting the stent area to the corresponding lunimal references without damage to the platform.
Table 1. Characteristics of drug-eluting stents
Stent | Plataform | Polymer/ coating | Drug | Strut thickness (µm) | Maximum expansion (mm) | Studies with 1 month DAPT duration |
---|---|---|---|---|---|---|
XIENCE | Cobalt-chromium | Permanent/ circumferential | Everolimus | 81 | 2-3.25 → 3.75 3.5-4 → 5.5 |
Xience 28 USA (NCT03815175) |
SYNERGY | Platinum-chromium | Bioresorbible/ abluminal | Everolimus | 74 | 2.25 → 3.5 3-3.5 → 4.25 4-5 → 5.75 |
SENIOR (NCT02099617) |
Onyx | Nickel-platinum-chronium | Permanent/ circumferential | Zotarolimus | 81 | 2.5-3 → 3.75 3.5-4 → 4.75 4.5-5 → 5.75 |
Onyx ONE (NCT03344653) |
Orsiro | Cobalt-chromium | Bioresorbible/ circumferential | Sirolimus | 60 | 2.5-3 → 3.5 3.5-4 → 4.5 |
Bioflow-DAPT (NCT04137510) |
Ultimaster Tansei | Cobalt-chromium | Bioresorbible/ abluminal | Sirolimus | 80 | 2.25-3 → 4.5 3.5-4 → 5.5 |
Master DAPT (NCT03023020) |
BioFreedom | Stainless-steel | Polymer-free/ abluminal | Umirolimus | 112 | 2.5-3 → 4.76 3.5-4 → 5.96 |
LEADERS FREE (NCT01623180) |
Angiolite | Cobalt-chromium | Permanent/circumferential | Sirolimus | 75-85 | 2-2.5 → 4 2.75-3.5 → 5.25 4-4.5 → 6 |
– |
DAPT, dual antiplatelet therapy. |
Polymers are the reservoir from which the drug is released in a controlled way towards the arterial wall. XIENCE (Abbott), and Resolute Onyx (Medtronic) stents include permanent polymers, but with improved biocompatibility. The XIENCE polymer is highly fluorinated, which minimizes adhesion and platelet activation increasing the safety profile. The Onyx stent uses Biolinx that consists of a mixture of polymers with hydrophilic capabilities that make it biocompatible and hydrophobic for a prolonged and uniform drug release. However, despite the good results reported with the new permanent polymers, some companies have decided to develop bioresorbable polymers (SYNERGY [Boston Scientific], Orsiro [Biotronik], Ultimaster Tansei [Terumo] stents, etc.). The use of a bioresorbable polymer-based coating facilitates degradation after the drug complete release just leaving 1 bare-metal stent behind 3-4 months after implantation, which prevents the remaining polymer from participating in the immune response associated with stent thrombosis. The biodegradable polymer-based SYNERGY stent has shown a rate of stent thrombosis of 0% at the 6-month follow-up, and a rate of target lesion revascularization similar to the one observed with the permanent polymer-based Promus Element stent (Boston Scientific).4 On the other hand, the biodegradable polymer-based Orsiro stent had a significantly lower rate of target lesion failure compared to the XIENCE stent with permanent polymer.5 However, the XIENCE stent group had more and longer stents implanted, both factors associated with adverse events at follow-up.
Polymer-free stents stand as an alternative to biodegradable polymer-based stents. The most representative of the former is the BioFreedom (Biosensors). It consists of a stainless-steel platform with micropores that store biolimus for a 1-month release of the drug. The lack of a polymer may potentially allow to shorten the duration of the period on dual antiplatelet therapy down to 1 month, like with bare metal stents. In the LEADERS FREE trial,6 the BioFreedom was compared to a bare metal stent in patients with high-risk of bleeding and a 1-month course of dual antiplatelet therapy. The outcomes proved that it was superior regarding the primary evaluation criteria for the safety and efficacy profile. Afterwards, almost every company has conducted or started safety and efficacy trials with 1-month courses of dual antiplatelet therapy (table 1).
Therefore, several advances have been made with new generation DES. The clinical impact associated with strut thickness reduction or the selection of bioresorbable polymer-based stents vs permanent or polymer-free stents is still under discussion. However, these improvements have had significant repercussions in the stent navigability, overexpansion capabilities, and selection of duration for dual antiplatelet therapy. However, these stent properties highly demanded by interventional cardiologists should always be based on a robust set of trials that confirm the efficacy and safety profile of each stent. Currently, the Angiolite stent (iVascular) is in this stage. It has recently joined the family of DES. This stent is an ultra-thin strut (75 µm to 85 µm) cobalt-chromium platform made from a biostable fluorinated polymer plus sirolimus as the antiproliferative drug. In the ANCHOR trial it showed an excellent degree of endothelization on the optical coherence tomography,7 with an 83% strut endothelial coverage at 3 months. Afterwards, the ANGIOLITE trial compared it to the standard DES and found a 0.04 mm late luminal loss vs the 0,08 mm of the XIENCE stent, as well as a low rate of events at the 2-year follow-up in both groups8 (target lesion failure, 7.1% with the Angiolite vs 7.6% with the XIENCE). In an article published on REC Interventional Cardiology, Pérez de Prado et al.9 presented a multicenter real-world registry of patients treated with the Angiolite stent. This registry could be considered the «moment of truth» for this stent. We should remember that the Absorb stent (Abbott) had excellent immediate results and at the 5-year follow-up10 in the early trials. However, it showed a high rate of stent thrombosis when used in real-world patients. The ANCHOR registry included 646 patients with a 2-year clinical follow-up. A total of 30% of these patients were diabetics. ST-segment elevation myocardial infarction was the clinical presentation in almost 25% of these patients, and nearly 50% of them had multivessel disease. The rate of target vessel failure and the rate of stent thrombosis at the 2-year follow-up were 3.4%, and 0.9%, respectively. In this sense, the stent offers similar results to those obtained with state-of-the-art DES as the table of the supplementary data of the aforementioned article shows.9 However, these promising results will need to be confirmed at the 5-year follow-up. The behavior of the stent in the most complex lesions like bifurcations or chronic total occlusions associated with worse clinical outcomes—underrepresented in the registry—also needs to be assessed.
FUNDING
The authors received no funding whatsoever while preparing this manuscript.
CONFLICTS OF INTEREST
J. Suárez de Lezo has received fees from Terumo for his role as a consultor for Abbott and AstraZeneca, as well as for his presentations in training courses and seminars. P. Martín received fees from Cathmedical for his role as a consultor, as well as from Abbott and Boston Scientific for his presentations in training courses and seminars.
REFERENCES
1. Moses JW, Leon MB, Popma JJ, et al.;SIRIUS Investigators. Sirolimus-eluting stents versus standard stents in patients with stenosis in a native coronary artery. N Engl J Med. 2003;349:1315-1323.
2. Stone GW, Ellis SG, Cox DA, et al.;TAXUS-IV Investigators. A polymer-based, paclitaxel-eluting stent in patients with coronary artery disease. N Engl J Med. 2004;350:221-231.
3. Camenzind E, Steg PG, Wijns W. Stent thrombosis late after implantation of first-generation drug-eluting stents:a cause for concern. Circulation. 2007;115:1440-1455.
4. Meredith IT, Verheye S, Dubois CL, et al. Primary endpoint results of the EVOLVE trial:a randomized evaluation of a novel bioabsorbable polymer-coated, everolimus-eluting coronary stent. J Am Coll Cardiol. 2012:59:1362-1370.
5. Kandzari DE, Mauri L, Koolen JJ, et al. Ultrathin, bioresorbable polymer sirolimus-eluting stents versus thin, durable polymer everolimus-eluting stents in patients undergoing coronary revascularisation (BIOFLOW V):a randomised trial. Lancet. 2017;390:1843-1852.
6. Urban P, Meredith IT, Abizaid A, et al. Polymer-free drug-coated coronary stents in patients at high bleeding risk. N Engl J Med. 2015;373:2038-2047.
7. Puri R, Otaegui I, Sabate M, et al. Three- and 6-month optical coherence tomographic surveillance following percutaneous coronary intervention with the Angiolite(R) drug-eluting stent:The ANCHOR study. Catheter Cardiovasc Interv. 2018;91:435-443.
8. Moreu J, Moreno-Gomez R, Perez de Prado A, et al. First-in-man randomised comparison of the Angiolite durable fluoroacrylate polymer-based sirolimus-eluting stentversus a durable fluoropolymer-based everolimus-eluting stentin patients with coronary artery disease:the ANGIOLITE trial. EuroIntervention. 2019;15:e1081-e1089.
9. Pérez de Prado A, Ocaranza-Sánchez R, Lozano Ruiz-Poveda F, et al. Real-world registry of the durable Angiolite fluoroacrylate polymer-based sirolimus-eluting stent:the EPIC02–RANGO study. REC Interv Cardiol. 2021. https://doi.org/10.24875/RECICE.M21000223.
10. Onuma Y, Dudek D, Thuesen L, et al. Five-Year Clinical and Functional Multislice Computed Tomography Angiographic Results After Coronary Implantation of the Fully Resorbable Polymeric Everolimus-Eluting Scaffold in Patients With De Novo Coronary Artery Disease:The ABSORB Cohort A Trial. JACC Cardiovasc Interv. 2013;6:999-1009.
* Corresponding author: Servicio de Cardiología, Hospital Universitario Reina Sofía, Menéndez Pidal s/n, 14004 Córdoba, Spain.
E-mail address: jslht@yahoo.es (J. Suárez de Lezo).
Transcatheter aortic valve implantation (TAVI) has become a widely used therapeutic strategy to treat symptomatic severe aortic stenosis. Certain randomized clinical trials available have already described the prognostic benefit of this technique in elderly patients with high or very high surgical risk. Also, when implanted via transfemoral access, it has proven non-inferior or even superior compared to surgical aortic valve replacement in low- and intermediate-risk patients. Therefore, the current recommendations support its use in elderly patients with severe aortic stenosis regardless of their surgical risk.1
However, since it is an age-related heart valve disease without an effective medical therapy yet, the prevalence of severe aortic stenosis has been growing parallel to life expectancy. As a matter of fact, this disease has huge repercussions in the patients’ survival rate and quality of life.2 Consequently, nonagenarian patients with severe aortic stenosis are a group in continuous expansion, and, to this date, the best way to treat them is still under discussion. Since most of these patients have traditionally been misrepresented in the clinical trials and there are registries with good results but a possible selection bias, the decision to treat these patients with TAVI is still challenging and is made on an individual basis after meticulous assessment of the patients by the heart team.
Data from the STS/ACC TVT registry on the results of 3773 patients ≥ 90 age provide us with relevant information on this clinical setting.3 Data show a higher mortality rate compared to younger patients at 30 days (8.8% vs 5.9%; P < .001) and 1 year (24.8% vs 22.0%; P < .001) mainly due to higher rates of in-hospital major bleeding (8.1% vs 6.8%; P < .001) and strokes (2.7% vs 2.1%; P = .021). Table 1 shows the data from the main international registries on nonagenarian patients treated with TAVI.
Table 1. Data of nonagenarian patients treated with TAVI from the main international registries
Study, year | N (≥ 90 years) | Procedural success | Major bleeding | 30-day mortality rate | 1-year mortality rate |
---|---|---|---|---|---|
SwissTAVI,4 2021 | 507 | N/A | 13% | 6.7% | 19.7% |
Mentias et al.,5 2019 | 13 544 | N/A | 10% | 3.6% | 26.6% |
Vlastra et al.,6 2019 | 882 | N/A | 8% | 9.9% | N/A |
Doshi et al.,7 2018 | 1163 | N/A | 35% | 6.0% | N/A |
Elgendy et al.,8 2018 | 5840 | N/A | 28% | 6.6% | N/A |
McNeely et al.,9 2017 | 3531 | N/A | 34% | 8.4% | 25.4% |
STS/ACC TVT,3 2016 | 3773 | N/A | 8% | 8.8% | 24.8% |
N/A, not available; TAVI, transcatheter aortic valve implantation. |
In a study recently published on REC: Interventional Cardiology, Cepas-Guillén et al. describe the national experience with TAVI in nonagenarian patients with severe aortic stenosis between 2009 and 2018.10 The findings were compared to those from patients between 75 and 90 years who received the same treatment during the same period of time. A total of 8073 patients—387 nonagenarians and 7686 patients between 75 and 90 years—were included. The authors saw a higher in-hospital mortality rate in nonagenarian patients without significant differences at the 1-year follow-up (although with a tendency towards a higher mortality rate in patients ≥ 90 years). In the multivariate analysis, age was not significantly associated with a higher all-cause mortality rate, but the presence of comorbidities such as atrial fibrillation or worsening renal function. Also, a higher surgical risk was reported.10 The role of comorbidities in nonagenarian patients was analyzed by a former substudy that included 117 consecutive patients around 90 years old (median age, 91.1 years; 117 women) from the PEGASO (Prognosis of symptomatic severe aortic stenosis in octogenarians) and IDEAS (Influence of the severe aortic stenosis diagnosis) registries conducted in our country.11 A high comorbidity burden, characterized by scores ≥ 3 in the Charlson comorbidity index, present in a high number of patients, was associated with a high mortality rate at the 1-year follow-up.
Nonetheless, data from the SwissTAVI registry confirmed a tendency towards higher rates of mortality, stroke, and pacemaker implantation in the elderly group (< 70, 70-79, 80-89, and > 90 years) among the 7097 patients treated with TAVI between 2011 and 2018 (median age, 82 ± 6.4 years; 49.6% women) in Switzerland.4 It is interesting that older age was associated with a lower standard mortality rate compared to the general population of the same group without any differences reported among nonagenarian patients. Same as in the study conducted by Cepas-Guillén et al.,10 the mean comorbidity rate of nonagenarian patients included in this registry was lower compared to that of patients < 90 years, suggestive that it is a highly selected population, which is unequivocally associated with a better prognosis in the short- and long-term. Despite of this, in this series, vascular complications and major bleeding increased significantly among nonagenarian patients. In the metanalysis conducted by Sun et al.,12 the rate of major bleeding reported was similar above and below the 90-year mark with a relative risk of 1.17 (95% confidence interval, 1.04-1.32). On the contrary, the rate of vascular complications went up > 90 years, especially when non-femoral access techniques were used. In the Spanish national series, transfemoral access was more frequently used in patients < 90 years. Although no random comparison of the access routes was conducted, most data support the idea that risks are higher when the non-femoral access is used.13 Also, these data recommend the use of this access in elderly patients whenever possible. Also, a tendency towards better results has been confirmed as heart teams have been gaining experienced in the management of this group of patients, both in the selection and implantation technique used as well as in further approaches with lower rates of complications and 30-day mortality > 50% at the 4-year follow-up.5 Noteworthy, it would be interesting to have more data on the treatment received by the patients, especially regarding antiplatelet therapy, given these patients’ higher risk of bleeding with the use of 2 different antiplatelet drugs, instead of 1,14 and a longer follow-up too.
Added to all this, we should recognise not only the presence of comorbidities, but also frailty, and other geriatric symptoms—prevalent all of them—that have a significant prognostic impact in elderly patients treated with TAVI.15 Different scales have been described, some of them very easy to implement and based on easy parameters like the presence of lower-limb frailty, cognitive impairment, anemia, and hypoalbuminemia, which allow us to predict mortality at the 1-year follow-up.16 The implementation of measures is essential to reverse frailty—if present—and prevent the appearance of delirium and other complications during admission since this improves the prognosis of patients significantly.
Finally, we wish to congratulate the Interventional Cardiology Association of the Spanish Society of Cardiology Association for their remarkable work in this area—in continuous expansion and growth—since the experience and learning gained on this regard will contribute to improve the treatments that we will administer to our patients.
FUNDING
No funding whatsoever.
CONFLICTS OF INTEREST
None declared.
REFERENCES
1. Baumgartner H, Falk V, Bax JJ, et al.;ESC Scientific Document Group. 2017 ESC/EACTS Guidelines for the management of valvular heart disease. Eur Heart J. 2017;38:2739-2791.
2. Osnabrugge RLJ, Mylotte D, Head SJ, et al. Aortic stenosis in the elderly:disease prevalence and number of candidates for transcatheter aortic valve replacement:a meta-analysis and modeling study. J Am Coll Cardiol. 2013;62:1002-1012.
3. Arsalan M, Szerlip M, Vemulapalli S, et al. Should transcatheter aortic valve replacement be performed in nonagenarians?:insights from the STS/ACC TVT registry. J Am Coll Cardiol. 2016;67:1387-1395.
4. Attinger-Toller A, Ferrari E, Tueller D, et al. Age-Related Outcomes After Transcatheter Aortic Valve Replacement:Insights From the SwissTAVI Registry. JACC Cardiovasc Interv. 2021;14:952-960.
5. Mentias A, Saad M, Desai MY, et al. Temporal Trends and Clinical Outcomes of Transcatheter Aortic Valve Replacement in Nonagenarians. J Am Heart Assoc. 2019;8:e013685.
6. Vlastra W, Chandrasekhar J, Vendrik J, et al. Transfemoral TAVR in Nonagenarians:From the CENTER Collaboration. JACC Cardiovasc Interv. 2019;12:911-920.
7. Doshi R, Patel V, Shah P. Comparison of in-hospital outcomes between octogenarians and nonagenarians undergoing transcatheter aortic valve replacement:a propensity matched analysis. J Geriatr Cardiol. 2018;15:123-130.
8. Elgendy IY, Mahmoud AN, Elbadawi A, et al. In-hospital outcomes of transcatheter versus surgical aortic valve replacement for nonagenarians. Catheter Cardiovasc Interv. 2019;93:989-995.
9. McNeely C, Zajarias A, Robbs R, Markwell S, Vassileva CM. Transcatheter Aortic Valve Replacement Outcomes in Nonagenarians Stratified by Transfemoral and Transapical Approach. Ann Thorac Surg. 2017;103:1808-1814.
10. Cepas-Guillén PL, Regueiro A, Sanmiguel Cervera D, et al. Outcomes of nonagenarians after transcatheter aortic valve implantation. REC Interv Cardiol. 2021. https://doi.org/10.24875/RECICE.M21000220.
11. Bernal E, Ariza-SoléA, Formiga F, et al.;Influencia del Diagnóstico de Estenosis Aórtica Severa (IDEAS) Registry Investigators. Conservative management in very elderly patients with severe aortic stenosis:Time to change?J Cardiol. 2017;69:883-887.
12. Sun Y, Liu X, Chen Z, et al. Meta?analysis of predictors of early severe bleeding in patients who underwent transcatheter aortic valve implantation. Am J Cardiol. 2017;120:655?661.
13. Drudi LM, Ades M, Asgar A, et al. Interaction between frailty and access site in older adults undergoing transcatheter aortic valve replacement. JACC Cardiovasc Interv. 2018;11:2185?2192.
14. Sanz-Sánchez J, Pivato CA, Leone PP, et al. Tratamiento antiagregante plaquetario único o doble tras implante percutáneo de válvula aórtica. Metanálisis de ensayos clínicos aleatorizados. REC Interv Cardiol. 2021. https://doi.org/10.24875/RECIC.M21000206.
15. Díez-Villanueva P, Arizá-SoléA, Vidán MT, et al. Recommendations of the Geriatric Cardiology Section of the Spanish Society of Cardiology for the Assessment of Frailty in Elderly Patients With Heart Disease. Rev Esp Cardiol. 2019;72:63-71.
16. Afilalo J, Lauck S, Kim DH, et al. Frailty in Older Adults Undergoing Aortic Valve Replacement:The FRAILTY-AVR Study. J Am Coll Cardiol. 2017;70:689-700.
* Corresponding author: Servicio de Cardiología, Hospital Universitario de La Princesa, Diego de León 62, 28006 Madrid, Spain.
E-mail address: pablo_diez_villanueva@hotmail.com (P. Díez-Villanueva).
Coronary artery disease (CAD) is one of the leading causes of death worldwide. These rates are only likely to be buoyed in the future by the rising prevalence of obesity, diabetes, and metabolic syndrome.
The pathogenesis of atherosclerosis, the main cause of CAD, has been a topic of great interest.1 As a brief review, dyslipidemia plays a key role. Beginning with the incorporation of serum low-density lipoprotein into the endothelium tunica intima, chemokines and endothelial adhesion molecules attract macrophages into the arterial wall. Eventually, cholesterol droplets are incorporated into the macrophage cytosol, become oxidized, and form the so-called ‘foam cell’. In a reciprocal exchange, inflammatory mediators released by foam cells trigger ongoing endothelial damage, fibrosis, and intimal hyperplasia. Eventually, coronary artery stenosis occurs; a condition at the basis of CAD and for which inflammation is a key component.
Inflammation is likely to play a role in both stable and unstable CAD. Many patients may present small atheromas, but still suffer from acute coronary thromboses–the acute coronary syndrome. Pathological studies have shown that T-cells, macrophages, and mast cells congregate to plaque rupture sites where they upregulate matrix metalloproteases, degrade collagen, and weaken the fibrous cap that supports the plaques. Additionally, once the endothelium is exposed to the plaque following a rupture, these inflammatory cells facilitate thrombosis, and platelet plug formation. As clues to their damage, such patients show high levels of interleukin-6, and C-reactive protein in their blood.1
ANTI-INFLAMMATORY THERAPIES FOR CAD
The centerpiece role of inflammation in CAD led to descriptions of atherosclerosis as a “chronic inflammation of the arteries,” a fact borne out by science as early as the 1980s.2 Yet, decades later no routinely used CAD therapies target any inflammatory pathways (figure 1). Many extant anti-inflammatory therapies have tried so but have proven unsuccessful. For instance, corticosteroids exhibit a broad range of anti-inflammatory properties, but their promotion of dyslipidemia and hypertension ultimately cause atherogenesis. Non-steroidal anti-inflammatory drugs other than aspirin inhibit prostacyclin, which increases vascular tone and platelet aggregation in the coronary arteries. Interest in methotrexate arose from observational studies that showed cardioprotective properties in patients with rheumatoid arthritis. However, a randomized clinical trial that analyzed its effect in patients with atherosclerosis showed that it does not lower the serum inflammatory markers or prevent myocardial infarction.3
Figure 1. Therapeutic foundations for the management of coronary artery disease. The figure shows agents with proven mortality benefits in the management of coronary artery disease. Although thrombosis, dyslipidemia, and inflammation are all key in the pathogenesis of coronary artery disease, the scarcity of effective anti-inflammatory agents is evident.
An interleukin-1b inhibitor, canakinumab, was the first agent to successfully prevent cardiovascular events in patients with a recent myocardial infarction. In the CANTOS trial, canakinumab 150 mg once daily lowered the rates of nonfatal myocardial infarction, stroke, and death (hazard ratio [HR], 0.85; 95% confidence interval [95%CI], 0.74-0.98) at the cost of increasing the rate of fatal sepsis, and infection (0.31 vs 0.18 per 100 person-years; P = .02). This trial was an important scientific breakthrough that showed that the inflammatory hypothesis is a therapeutic option for CAD. However, the costs of therapy and a modest effect size have both limited its use.4
Like methotrexate, interest in colchicine was borne out by observational data. In patients suffering gout flares, the use of colchicine was associated with fewer cardiovascular events compared to non-use.5 These observations led to a non-blinded randomized analysis of colchicine (the Low-dose colchicine for secondary prevention of cardiovascular disease [LoDoCo] trial) that proved a reduction in cardiovascular events in patients with stable CAD.6 Although there were some methodological limitations, the trial served as the niche for more upcoming robust clinical data on colchicine.
Colchicine is an inhibitor of microtubules and may prevent leukocyte migration into sites of plaque formation and rupture. Moreover, colchicine helps inhibit the formation of the NLRP3 inflammasome –a structure recently involved in cytokine mediated cell death. These pro-inflammatory protein complexes are activated by cholesterol crystals in macrophages and secrete interleukin (IL)-1b, the cytokine target of canakinumab. Colchicine has also been shown to reduce C-reactive protein, IL-1, and IL-6.7
A larger follow up trial to the initial LoDoCo trial, the LoDoCo2, enrolled 5522 patients with chronic CAD, and randomized them to receive colchicine or placebo for a median of 28.6 months after an open label run-in period to ensure colchicine tolerability. Colchicine was associated with a 31% reduction (HR, 0.69; 95%CI, 0.57-0.83; P < .001) of cardiovascular death, infarction, ischemic stroke, and revascularization. Adversely, patients who received colchicine showed higher rates of non-cardiovascular death, although the rate of events was low (HR, 1.51; 95%CI, 0.99-2.31; P = .06).8 Other adverse occurrences and intolerances were rare.
COLCHICINE IN ACS
Another 2 trials examined colchicine in a recent myocardial infarction setting: the COLCOT trial (efficacy and safety profile of low doses of colchicine after myocardial infarction), and the COPS trial (colchicine in patients with acute coronary syndrome). The first and larger of the two, the COLCOT trial, randomized 4745 patients who had a myocardial infarction within 30 days into colchicine therapy or placebo and followed them for a median of 22.6 months. The patients who received colchicine enjoyed a 23% reduction (HR, 0.77; 95%CI, 0.61-0.97; P = .02) in the composite endpoint of cardiovascular death, cardiac arrest, ischemic stroke, infarction, and angina requiring urgent revascularization. Though a positive outcome, it was driven primary by fewer revascularizations in the colchicine arm. Additionally, patients randomized to colchicine experienced more pneumonia (0.9% vs 0.4%; P = .03) compared to placebo.9 An early administration of colchicine was associated with greater benefits (within 3 days) in the COLCOT trial (HR, 0.52; 95%CI, 0.32-0.84 for initiation within 3 days vs HR, 0.98; 95%CI, 0.53-1.75 for initiation between days 4 and 7).10
The COPS trial enrolled 795 patients and randomized them to receive colchicine or placebo for 12 months. At the 1-year follow-up, no statistical differences were seen in the composite endpoint of cardiovascular death, infarction, ischemic stroke, and cardiac arrest with colchicine compared to placebo (HR, 0.65; 95%CI, 0.38-1.09; P = .10), likely due to a lower rate of events from a shorter follow-up period, and a smaller sample size. Moreover, 8 patients from the colchicine arm and 1 from the placebo group died, an effect that reached statistical significance and was driven by non-cardiovascular mortality (P = .047).11 Although this trial enrolled relatively few patients, it reproduced a signal towards mortality as seen on the larger LoDoCo2 trial.
The largest trial of colchicine post-myocardial infarction, the Colchicine and spironolactone in acute MI (CLEAR SYNGERY, NCT03048825), is underway. It will randomize 7000 patients and help solve the issue of whether colchicine increases non-cardiovascular mortality. The findings of the COPS and LoDoCo2 trials may be spurious findings, similar to what was found in earlier statin trials, and eventually disproved. On the other hand, there could be an important impact of colchicine due to a reduction of host defenses that went previously unnoticed.
Colchicine may be the first widely available, inexpensive anti-inflammatory therapy for the management of CAD. However, the issue of non-cardiovascular mortality should be resolved before it is widely adopted.
FUNDING
The authors received no specific funding for this work.
CONFLICTS OF INTEREST
S.S. Jolly reports grant support from Boston Scientific and the Canadian Institutes of Health Research. W. Hijazi declared no conflicts of interest.
REFERENCES
1. Bouabdallaoui N, Tardif JC, Waters DD, et al. Time-to-treatment initiation of colchicine and cardiovascular outcomes after myocardial infarction in the Colchicine Cardiovascular Outcomes Trial (COLCOT). Eur Heart J. 2020;41:4092-4099.
2. Butt AK, Cave B, Maturana M, Towers WF, Khouzam RN. The Role of Colchicine in Coronary Artery Disease. Curr Probl Cardiol. 2021;46:100690.
3. Hansson GK. Inflammation, Atherosclerosis, and Coronary Artery Disease. N Engl J Med. 2005;352:1685-1695.
4. Nabel EG, Braunwald E., 2012. A Tale of Coronary Artery Disease and Myocardial Infarction. N Engl J Med. 2012;366:54-63.
5. Nidorf SM, Eikelboom JW, Budgeon CA, Thompson PL. Low-Dose Colchicine for Secondary Prevention of Cardiovascular Disease. J Am Coll Cardiol. 2013;61:404-410.
6. Nidorf SM, Fiolet ATL, Mosterd A, et al.;LoDoCo2 Trial Investigators. Colchicine in Patients with Chronic Coronary Disease. N Engl J Med. 2020;383:1838-1847.
7. Ridker PM, Everett BM, Pradhan A, et al.;CIRT Investigators. Low-Dose Methotrexate for the Prevention of Atherosclerotic Events. N Engl J Med. 2019;380:752-762.
8. Ridker PM, Everett BM, Thuren T, et al.;CANTOS Trial Group Antiinflammatory Therapy with Canakinumab for Atherosclerotic Disease. N Engl J Med. 2017;377:1119-1131.
9. Solomon D, Liu CC, Kuo IH, Zak A, Kim SC. Effects of colchicine on risk of cardiovascular events and mortality among patients with gout:a cohort study using electronic medical records linked with Medicare claims. Ann Rheum Dis. 2016;75:1674-1679.
10. Tardif JC, Kouz S, Waters DD, et al. 2019. Efficacy and Safety of Low-Dose Colchicine after Myocardial Infarction. N Engl J Med. 2019;381:2497-2505.
11. Tong DC, Quinn S, Nasis A, et al. 2020. Colchicine in Patients With Acute Coronary Syndrome. Circulation. 2020;142:1890-1900.
Corresponding author: Room C3-118, DBCVSRI Building, Hamilton General Hospital, 237 Barton St East, Hamilton, ON L8L 2X2, Canada.
E-mail address: sanjit.jolly@phri.ca (S.S. Jolly).
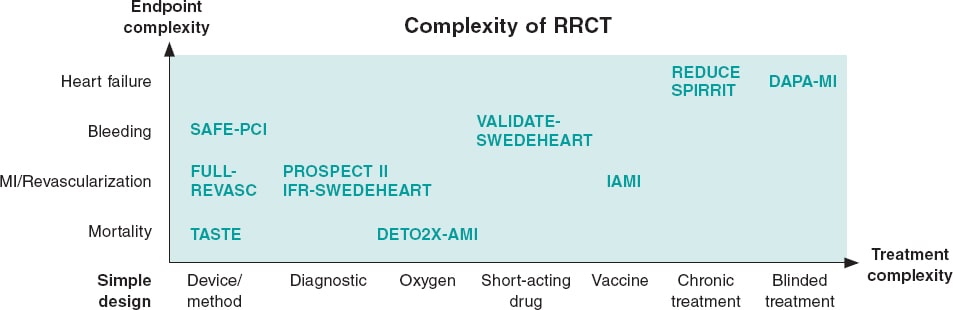
INTRODUCTION
The randomized clinical trial (RCT) has become the gold standard for evaluating clinical treatments thanks to its low selection bias and unknown confounders. However, good clinical practice guidelines and demands from regulatory agencies have become so elaborate over time that, basically, only big pharmaceutical companies have the resources to conduct large RCTs. Therefore, important questions raised by academic scientists could be impossible to test in clinical trials.
One way to circumvent these problems is to use the registry-based randomized clinical trial (RRCT) design. A RRCT uses the platform of an already-existing high-quality observational health registry as a case-report form for randomization and follow-up purposes. This design facilitates the randomization of a large number of patients over a short period of time, reduces costs to a fraction of the cost of conventional randomized clinical trials, and facilitates the follow-up of all eligible patients not enrolled in the study (table 1).1-4
Table 1. Major functions for trial conduction provided by the registry
Major functions for trial conduction provided by the registry |
Identification of eligible patients |
Alert investigator of an eligible patient |
Link to randomization module |
Randomization |
Collection of baseline and procedural characteristics from a registry (eCRF) |
Presentation of additional trial-specific questions for eCRF |
Identification of clinical endpoints (endpoint detection) |
Clinical outcomes reporting |
Reporting of characteristics of enrolled and non-enrolled patients from the overall population |
eCRF, electronic case report form. |
RRCT-SUITABLE REGISTRIES
Nearly all healthcare data are stored digitally today, which poses an excellent opportunity to use these data in a RRCT. However, healthcare records are often not structured in a way that allows useful data extraction. Today, disease-specific quality registries with full nationwide coverage are the most suitable ones as the basis for RRCTs, but this may change in the future. Our experience comes from using the Swedish Web-system for the Enhancement and Development of Evidence-based care in Heart disease Evaluated According to Recommended Therapies (SWEDEHEART) and its Swedish Coronary Angiography and Angioplasty Registry (SCAAR)5 through which a large number of RRCTs have been conducted or are in ongoing phases (table 2).6-10 The validation of registry data vs health records has an overall percent agreement of 96%.11 The first pure RRCT was the TASTE trial where thrombus aspiration in patients with ST-segment elevation myocardial infarction (STEMI) was studied with mortality as the primary endpoint.1 A large number of patients were rapidly included and with a limited budget by only using registries of baseline demographics, randomization, and endpoint collection in a prospective and randomized fashion. In this trial of a simple device intervention and a solid endpoint, the SWEDEHEART registry provided all the necessary steps to conduct a RCT (table 1). First, identify eligible patients and “flag” them with a pop-up window to the investigator appointed prior to the procedure. Secondly, open up a randomization window with 2 questions: Have the inclusion/exclusion criteria been met? Has the patient given his consent to enter the study? If the answers to both these questions were positive, the patient was randomized and the result shown on the screen momentarily. Thirdly, both the baseline characteristics and the follow-up endpoints were collected from the registry. Furthermore, data on all of the unrecruited patients with complete baseline characteristics are collected. It is interesting to compare the TASTE to the TOTAL trial that examined thrombus aspiration using the traditional RCT design.12 While the cost of the TOTAL trial was approximately €15 000 000 with 87 centers enrolling patients for 48 months on a 6-month follow-up, the cost of the TASTE trial was €500 000 (3%!) with 30 centers enrolling patients for 33 months on a 42-month follow-up being the results nearly identical. In these circumstances of low complexity in both treatment and endpoints an RRCT is superior, in almost every aspect, to a traditional RCT.
Table 1. Registry-based randomized clinical trials in the SWEDEHEART registry: completed, ongoing or in the pipeline
RRCT | Patients, N | Question | Dates |
---|---|---|---|
TASTE, Fröbert et al.1 (2013) | 7200 | Thrombus aspiration in primary PCI | 2013 + 2014 |
IFR-SWEDEHEART, Gotberg et al.6 (2017) | 2018 | iFR vs FFR in stable angina or ACS | 2017 |
VALIDATE-SWEDEHEART Erlinge et al.7 (2017) | 6006 | Bivalirudin vs UFH for PCI in ACS | 2017 |
DETO2X-AMI, Hofmann et al.8 (2017) | 6629 | Oxygen therapy in MI | 2017 |
FULL-REVASC, NCT02862119 | 4052 | FFR-guidance in MI | Enrollment stopped after 1545 patients |
PROSPECT-II, NCT02171065 | 900 | Near-infrared spectroscopy in PCI | Presented, TCT 2020 |
IAMI, Fröbert ret al.9 (2017) | 4400 | Influenza vaccination after MI | Completed enrollment |
SPIRRIT, NCT02901184 | 3200 | Spironolactone for HFpEF | Ongoing |
REDUCE, NCT03278509 | 6600 | Beta-blocker post MI in patients with ejection fraction > 50% | Ongoing |
ABC-AF, NCT03753490 | 6500 | Biomarker score-based treatment vs standard care | Ongoing |
MINOCA-BAT, NCT03686696 | 2048 | ACEi/beta-blockers after MI with non-obstructive CAD | Ongoing |
TACSI, NCT03560310 | 2200 | Post-CABG ACS, ticagrelor | Ongoing |
SWEDEGRAFT, NCT03501303 | 902 | CABG grafting | Completed enrollment |
Infinity-Swedeheart, NCT04562805 | 2400 | Disengaging DES vs DES | Ongoing |
DAPA-MI, NCT04564742 | 6400 | SGLT2 inhibitor post-AMI | Ongoing |
HELP-SWEDEHEARTa | 20 000 | Helicobacter pylori screening after AMI to prevent upper gastrointestinal bleeding (cluster-randomization) | Q2, 2021 |
SWITCHb | 20 000 | Prasugrel or ticagrelor post-MI (cluster-randomization) | In the pipeline |
BROKEN-Swedeheart, NCT04666454 | 1000 | Optimal medical treatment for Tako-tsubo syndrome | In the pipeline |
a Still unregistered; pilot study: NCT04289012. b Still unregistered. ACEi, angiotensin-converting enzyme inhibitor; AMI, acute myocardial infarction; ACS, acute coronary syndromes; CABG, coronary artery bypass graft; CAD, coronary artery disease; DES, drug-eluting stent; FFR, fractional flow reserve; HFpEF, heart failure with preserved ejection fraction; iFR, instantaneous wave-free ratio; MI, myocardial infarction; EFPCI cabg SGLT2, sodium-glucose cotransporter-2; PCI percutaneous coronary intervention; TCT, Transcatheter Cardiovascular Therapeutics conference; UFH, unfractionated heparin. |
ADVANTAGES AND LIMITATIONS OF PURE RRCTs COMPARED TO TRADITIONAL RCTs
The major advantages of the RRCT design are: a) a broader and more representative population to clinical reality; in the TASTE and VALIDATE-SWEDEHEART trials 70% of all eligible patients were included1,7; b) clinically significant endpoints were included, and not multiple composite weak or surrogate endpoints; c) long-term follow-up periods, actually life-long follow-ups, if applicable; d) thanks to random selection, bias and confounding factors are reduced to a minimum; e) significantly lower costs; f) rapid inclusion of a large number of patients; and g) initiated and conducted by independent academic researchers with no links to the industry.
The limitations are: a) open label design with a risk of biased endpoint reporting; b) rare, unexpected events may be missing, and serious adverse event reporting may be difficult; c) events are, for the most part, not adjudicated, which may result in variable data quality; d) difficulties having central chemical analysis and biobanking; e) long-term oral drugs can be difficult to distribute and follow; and f) lack of or limited site monitoring (figure 1).
Figure 1. Comparison of traditional randomized clinical trials (RCTs), registry studies, and registry-based randomized clinical trials (RRCT). Classical RCTs are the gold standard of clinical research, but they have limitations because they are very expensive, selective, and a cumbersome process. Retrospective registry studies can be conducted much cheaper, and may be more representative of the real world, yet they are always hampered by unknown confounders. An RRCT can profit from the best parts of these modalities. SAE, serious adverse event.
Depending on the limitations of the registry used, need for treatment escalation or endpoint complexity the RRCT can be complemented with different traditional trial elements resulting in a hybrid RRCT (figure 2).
Figure 2. Simple versus complex registry-based randomized clinical trials (RRCT). The purest RRCT examines a simple therapy like a thrombus aspiration device and has a robust endpoint like mortality. When the complexity increases for either treatment or endpoint, then additions to the RRCT design have to be made. These additions could be phone calls, central adjudication, or even blinded treatment with placebo. This increases complexity and costs, but the registry may still be the basis for the trial and facilitate performance. MI, myocardial infarction.
DEVELOPMENT OF RRCTs
In the SORT OUT series of coronary stent trials, baseline demographics and endpoint screening were conducted using a registry approach. However, randomization took place using different approaches (telephone allocation service, internet-based randomization systems), and endpoints were centrally adjudicated.13 In the SAFE-PCI study, randomization was performed outside the registry obtaining additional clinical information and adjudication to the registry data.14
In the VALIDATE-SWEDEHEART trial, 2 short-acting IV antithrombotic agents (bivalirudin and heparin) were assessed using the RRCT approach. As far as we know, this was the first pharmaceutical RRCT ever conducted.7 In a pharmaceutical trial the requirements from the medical regulatory authorities are more demanding even if the drugs have been approved and used for decades. Furthermore, we realized that our registry did not capture bleeding complications satisfactorily. Therefore, we added phone calls after 7 to 180 days followed by the central adjudication of bleeding complications and MI, limited serious event reporting, and data on the entire index hospitalization. Thanks to the simplicity of the trial, 25 centers were able to enroll over 6000 patients with MI over 2 years. Some large centers enrolled more than 1000 patients (figure 2, table 2).
In the IFR-SWEDEHEART trial the instantaneous wave-free ratio diagnostic modality was evaluated. The complexity of the intervention was low, but the composite endpoint included MI and unplanned revascularization.6 Although the endpoints were found in the registries, data were collected from medical records from the centers and adjudicated by a central committee.
In the DETO2X-AMI trial the endpoint was mortality, which does not need adjudication; however, the oxygen of the procedure had to be administered to the patient in a single blinded fashion adding some extra complexity to the study8 (figure 2, table 2). Similarly, the influenza vaccine study conducted post-MI (IAMI trial) needed blinded treatment.9 Furthermore, other countries without the SWEDEHEART registry structure were needed to get a sufficient number of patients, which resulted in a parallel randomization module and electronic case report forms (figure 2, table 2).9
There are 2 ongoing RRCTs with chronic oral treatment and a composite endpoint of death and hospitalizations due to heart failure: the REDUCE (beta-blocker post-MI, NCT03278509) and the SPIRRIT (Spironolactone for Heart Failure with Preserved Ejection Fraction, NCT02901184). However, despite the complex treatment and endpoint of both of them, they rely nearly only on registries. The treatment is randomized in the registry, prescribed, and then followed by the Swedish Prescribed Drug Register. Hospitalizations due to heart failure are collected from the National Patient Register where this diagnosis has proven to have a high validity in previous studies.
FUTURE POSSIBILITIES OF THE RRCT CONCEPT
So far, the RRCT technology has mostly been used for the assessment of devices or generic drugs that have been used for decades often with results that the treatment examined has been redundant, as it has been the case with the TASTE and VALIDATE-SWEDEHEART trials. Sometimes, as it occurred with the IFR-SWEDEHEART study, a new diagnostic procedure proves to be non-inferior to the current standard.6 This resulted in an IA recommendation for the instantaneous wave-free ratio in the clinical practice guidelines. In general, RRCTs have been deemed unsuitable by the medical regulatory authorities for first approval, but this is about to change. The INFINITY trial (NCT04562805) is examining a new type of stent capable of disengaging its metal struts after half a year. This is a currently ongoing RRCT whose objective is to support an approval given by the US Food and Drug Administration (FDA). Demographics, randomization, and endpoint follow-up are already taken care of by the SWEDEHEART registry, still a phone call at 1 month and 1 year combined with central adjudication was added.
The first study, that has been analyzing an expanded use for an oral drug, is the DAPA-MI study (NCT04564742) where dapagliflozin is being tested for post-MI patients with reduced ejection fraction but without diabetes. The registry is the basis of the study, yet visits have been added to dispense the blinded medication. The study is sponsored by AstraZeneca and intends to develop new more cost-efficient ways to conduct phase III trials. The study profits from 2 countries with nationwide MI registries, the UK and Sweden, with their MINAP16 and SWEDEHEART5 registries, respectively.
In Europe, the European Society of Cardiology has mostly relied on surveys to register different heart conditions. These are valuable, but they only give us a snapshot of a short timeframe and the selection of patients is unclear and may not be representative of the real world. However, a new initiative called EuroHeart17 has been trying to establish a common basic structure for continuous cardiac registries that could be used by any countries. One of its objectives is to facilitate conducting RRCTs in several European countries making the results more representative and allowing larger studies being conducted more rapidly.
CLUSTER-RANDOMIZED RRCTs
Cluster randomization design simplifies enrollment and does not often require signed informed consent forms, only general information about the ongoing study. It facilitates the recruitment of nearly all patients from a region during a certain period of time and it basicaly uses a cross-over design. In the HELP-SWEDEHEART trial—still not registered—20 000 patients diagnosed with MI in the SWEDEHEART registry will, based on hospital data, be cluster-randomized in a crossover design to receive Helicobacter pylori screening and, if they test positive, be recommended eradication therapy. The primary endpoint is upper gastrointestinal bleeding, which is collected from the National Patient Register. The still unregistered SWITCH trial is planning to investigate prasugrel compared to ticagrelor for the treatment of patients hospitalized due to MI with the composite endpoint of death, MI or stroke collected from the National Patient Register and the National Cause of Death Registry. A total of 4 Swedish regions will be randomized in blocks to standard use of either prasugrel or ticagrelor over 2 years.
In conclusion, RRCTs combine some of the best parts of the classical RCT design and traditional registries when conducting large, randomized, real-world, representative, and cost-effective clinical studies. They give academic researchers an opportunity to obtain important clinical answers that would have never been funded by industry.
FUNDING
The study is supported by the Swedish Heart and Lung Foundation, the Swedish Scientific Research Council, and the Knut and Alice Wallenberg Foundation. The author is solely responsible for the content of this manuscript.
CONFLICTS OF INTEREST
D. Erlinge declares having received speaker or advisory board fees from AstraZeneca, Bayer, Sanofi, and Chiesi.
REFERENCES
1. Fröbert O, Lagerqvist B, Olivecrona GK, et al. Thrombus aspiration during ST-segment elevation myocardial infarction. N Engl J Med. 2013;369:1587-1597.
2. James S, Frobert O, Lagerqvist B. Cardiovascular registries:a novel platform for randomised clinical trials. Heart. 2012;98:1329-1331.
3. Yndigegn T, Hofmann R, Jernberg T, Gale CP. Registry-based randomised clinical trial:efficient evaluation of generic pharmacotherapies in the contemporary era. Heart. 2018;104:1562-1567.
4. Lauer MS, D'Agostino RB, Sr. The randomized registry trial--the next disruptive technology in clinical research?N Engl J Med. 2013;369:1579-1581.
5. Start - SWEDEHEART (uu.se). Available at: https://www.ucr.uu.se/swedeheart/start-scaar. Accessed 8 Feb 2021.
6. Gotberg M, Christiansen EH, Gudmundsdottir IJ, et al. Instantaneous Wave-free Ratio versus Fractional Flow Reserve to Guide PCI. N Engl J Med. 2017;376:1813-1823.
7. Erlinge D, Omerovic E, Frobert O, et al. Bivalirudin versus Heparin Monotherapy in Myocardial Infarction. N Engl J Med. 2017;377:1132-1142.
8. Hofmann R, James SK, Jernberg T, et al. Oxygen Therapy in Suspected Acute Myocardial Infarction. N Engl J Med. 2017;377:1240-1249.
9. Fröbert O, Gotberg M, Angeras O, et al. Design and rationale for the Influenza vaccination After Myocardial Infarction (IAMI) trial. A registry-based randomized clinical trial. Am Heart J. 2017;189:94-102.
10. Hambraeus K, Held C, Johansson P, et al. SWEDEHEART Annual report 2012. Scan Cardiovasc J. 2014;48(Suppl 63):2-133.
11. Jernberg T, Attebring MF, Hambraeus K, et al. The Swedish Web-system for enhancement and development of evidence-based care in heart disease evaluated according to recommended therapies (SWEDEHEART). Heart. 2010;96:1617-1621.
12. Jolly SS, Cairns JA, Dzavik V. Primary PCI with or without Thrombectomy. N Engl J Med. 2015;373:682-683.
13. Raungaard B, Jensen LO, Tilsted HH, et al. Zotarolimus-eluting durable-polymer-coated stent versus a biolimus-eluting biodegradable-polymer-coated stent in unselected patients undergoing percutaneous coronary intervention (SORT OUT VI):a randomised non-inferiority trial. Lancet. 2015;385:1527-1535.
14. Rao SV, Hess CN, Barham B, et al. A registry-based randomized trial comparing radial and femoral approaches in women undergoing percutaneous coronary intervention:the SAFE-PCI for Women (Study of Access Site for Enhancement of PCI for Women) trial. JACC Cardiovasc Interv. 2014;7:857-867.
15. Neumann FJ, Sousa-Uva M, Ahlsson A, et al.;ESC Scientific Document Group. 2018 ESC/EACTS Guidelines on myocardial revascularization. Eur Heart J. 2019;40:87-165.
16. NICOR. Myocardial Ischaemia/MINAP (Heart Attack audit). Available at: https://www.nicor.org.uk/national-cardiac-audit-programme/myocardial-ischaemia-minap-heart-attack-audit/. Accessed 8 Feb 2021.
17. European Society of Cardiology. EuroHeart. Available at: https://www.escardio.org/Research/euroheart. Accessed 8 Feb 2021.
* Corresponding author: Department of Cardiology, Lund University, Skane University Hospital, S-221 85 Lund, Sweden.
E-mail address: david.erlinge@med.lu.se (D. Erlinge).
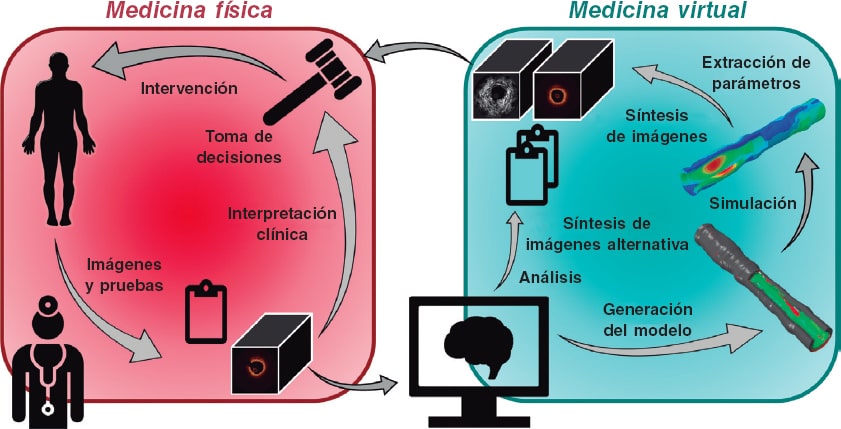
Medicine and technology have long advanced hand-in-hand. Clinicians push boundaries, expertly yielding the tools at their disposal. Scientists and engineers, in turn, respond to the demands and needs of clinicians by developing the next generation of tools, thereby expanding the space in which clinicians can operate and explore. This boundary-pushing partnership is particularly prevalent in the field of interventional cardiology, which has enthusiastically and effectively embraced advancements in percutaneous and imaging technologies to revolutionize cardiovascular medicine. Continuing this tradition, several developments in computational processing and modeling promise to enhance the utility and efficacy of arterial imaging, with some tools having already entered the clinical setting. For example, a tool that uses computational models built from computed tomography angiography to assess the fractional flow reserve has improved the clinical decision-making process and lowered the rates of unnecessary invasive procedures.1 Additional tools will further unify the physical and virtual realms of medicine through the bridge provided by imaging, offering both simple tools to label and quantify individual images and advanced tools to simulate and profile entire lesions. The future of the cooperative alliance between medicine and technology must be continuously nurtured and will continue to thrive with the enthusiastic and critical contributions of well-informed interventional cardiologists.
WHAT IS COMPUTATIONAL PROCESSING AND MODELING?
Computational processing is the application of algorithms and software to perform specified and encoded procedures. Computational processing can be applied to intravascular images to enhance, characterize or detect and quantify features depicted in the images. One application of such processing is to extract physiological features used to generate computational models of the imaged vessel region. Computational modeling is the creation and use of virtual representations of physical systems. Such representations can be programmed with sets of rules that prescribe how they should behave and respond under different conditions, and in that way the models can be used to simulate the behavior of the physical system under various hypothetical scenarios.
Unmet NEEDs IN INTERVENTIONAL CARDIOLOGY
In moving towards a more personalized and precise provision of medical care, the convergence of intravascular imaging with computational processing and modeling will be a pivotal step to empower interventional cardiologists. The role and need for this convergence (figure 1), part of a wider vision of computational cardiology,2 is highlighted by several key challenges faced by the current interventional cardiology practice.
Figure 1. Our vision for the future of cardiovascular medicine is one in which physical and virtual medicine forms a continuum. Clinicians collect data including images and other test results from a patient. Anatomical and morphological information will be automatically extracted by algorithmic processing routines, distilled into reported quantitative metrics, and used to generate patient-specific computational models. Various simulated tests and procedures will be performed on the virtual patient. Results of the analyses and simulations will be transformed back into clinical data to enable a seamless integration and assessment by the heart team; the outcomes could inform the decision-making process and guide the patient's procedure.
Standard assessments with less interventional workload
Among the most critical and urgent roles of computational processing is integrating and augmenting—not displacing—the role played by interventional cardiologists. Computational methods can remove the inter- and intra-observer variability, time consumption, and monotony associated with extensive manual measurements of intravascular images. Such analysis can be performed in the background and continuously without the constraints of busy clinical schedules. Support from processing technologies can also assist physicians with limited training, experience or expertise who may otherwise be unable to identify important features in intravascular images. Additionally, modeling offers relevant quantitative metrics of the vascular state that simply cannot be directly measured, such as estimates of stress along and within the vessel walls.
Patient profiling and stratification
The core premise of precision medicine is that patient populations can be segmented into narrow classes that respond differently to interventions. The cardiology community has proposed broad classifications to divide atherosclerotic plaques, typically driven by the prevailing tissue type and presumed degree of progression.3 Despite or in deference to its simplicity, there have been few significant updates to this classification in recent decades, even as intravascular imaging offers more and richer information on lesion geometry and morphology and as treatment of the disease has evolved. Computational processing and modeling may offer improved profiling of individual patients on the basis of clinical presentation, disease state, detailed lesion phenotype, and even mechanical condition. By computing series of quantitative measures to describe patient and lesion, the possibility of building a more robust patient profile becomes real. Such granular profiling could enable stratification to better assess who benefits the most from which interventions, thus guiding the therapeutic decisions.4
Prediction and risk assessments for clinical decision support
In addition to improved patient profiling and stratification, modeling offers the ability to engage in truly personalized risk assessment and prediction of disease progression under various treatment regimens. Because much of the interpretation of intravascular images is currently qualitative, decision-making during patient care can be an exquisite art and formulaic science alike and depend on each cardiologist’s personal experiences (and biases), training, and institutional practices. Advancing beyond personal clinician experience and intuition, computer processing and modeling offer repeatable, standardized quantification to inform the decision-making process. For example, simulations of detailed patient- and lesion-specific models, or “Digital Twins”,5 could facilitate various virtual interventions or intervention parameters to be tested before selecting an optimal strategy to minimize risk. Alternatively, disease progression and plaque growth models may help to predict which vessels and mild lesions may progress dangerously—suggesting the need for prophylactic action—and which are likely to remain benign and inconsequential over time.
TECHNOLOGICAL Tools in DEVELOPMENT
To fulfill the needs of computational processing and modeling in interventional cardiology, various new technologies are being developed that leverage the rich data available from intravascular imaging. The detection and measurement of geometric features is already available in limited cases, and it is likely to expand. The automated delineation of the lumen and external elastic lamina is incorporated in some intravascular ultrasound systems, and developments in computational processing have recently yielded promising results to identify these, as well as the internal elastic lamina, in optical coherence tomography images. Facilitated by automated detection of inner and outer vessel borders, automatic measurements from pullbacks such as lumen area, plaque burden, eccentricity, and remodeling index will reduce the need for manual identification and annotation of the most critical frames and will enable better visualization of diseased vessels. This information may also be used, for example, in the proper sizing of balloons and stents.
Advances in image processing also offer improved availability of information on lesion morphology. While experts are generally adept at determining the composition and distribution of plaque from cross-sectional images, doing so is a slow process that requires extensive expertise. Increased availability of automated virtual histology will improve the characterization, profiling, and stratification of lesion phenotypes. New image-based methods to characterize the stiffness of diseased tissue also promise greater insight into the mechanical profile of a lesion. Altogether, this information on plaque distribution and properties will help cardiologists to plan and guide interventions (eg, by informing the need for lesion preparation or modification prior to ballooning or stenting).
Computational modeling is a central focus of ongoing technological research and development. The ability to simulate disease progression and interventions is an enticing challenge that has been drawing the attention of multidisciplinary teams. Among these efforts, major collaborative, international European projects have sought to develop and refine advanced predictive models of atherosclerotic plaque processes and angioplasty by integrating patient risk factors, blood panel results, and imaging data.6 Robust longitudinal validation of such models remains an obstacle.
Computational processing offers another little-explored function to synthesize and enhance images. As intravascular imaging is the cornerstone of interventional cardiology, these generative abilities could be used with great effect to improve diagnostic image quality and efficacy, convey information generated from computational models, and facilitate education and training for reading and interpreting images.
Several technologies in development may require changes to future clinical practice. For example, some methods require multiple image pullbacks or simultaneous measurement of pressure and matched image acquisition. Changes will be limited by the corresponding progress in hardware development and adoption, demonstrated cost-benefit tradeoffs for patients, and receptiveness of the interventional cardiology field.
THE Indispensible ROLE OF INTERVENTIONAL CARDIOLOGISTS
Interventional cardiologists will not only have a pivotal role in the future adoption of computational processing and modeling technologies but also an important present role in defining and achieving that future. Those experienced in managing and treating patients are needed to direct, develop, and shepherd new technologies—their deep knowledge of the demands and practical limitations of clinical care are indispensable to scientists and engineers. There is also ongoing profound need for data with which to train and validate new methods and models. Here, too, the involvement, expertise, and contributions of collaborative cardiologists are essential.
The increasing integration of more complex technologies also introduces a growing imperative for cardiologists to further cultivate their technical literacy. While medical and health sciences must remain a priority in the training of interventional cardiologists, broader training is an important prerequisite to critically assess new claims and make informed decisions on the applicability and reliability of new techniques as they enter the medical arena. Clinicians will need to understand the assumptions, uncertainties, and conditions under which these tools should be beneficially applied to treat their patients. Interventional cardiologists and other medical professionals are already well-equipped for many of these tasks. Looking beyond the novelty and flair of the methods, familiar and fundamental concepts considered for other diagnostic tests, such as sensitivity and specificity, should be equally applied to scrutinize advanced new software tools.
As computational processing and modeling converge with intravascular imaging in the coming years, interventional cardiologists will be empowered to deliver more personalized and precise medical care. Those in the field should expect to play an active role in the development, assessment, and adoption of new technologies, and equip themselves with the evolving knowledge and skills necessary to make the most of these tools in the management of their patients.
FUNDING
This work was supported by the U.S. National Institutes of Health (Bethesda, MD, United States; grant number 5R01GM049039-24) and the Massachusetts Institute of Technology (Cambridge, MA, United States; MathWorks Engineering Fellowship).
CONFLICTS OF INTEREST
M. Olender and E.R. Edelman have the patent “Arterial Wall Characterization in Optical Coherence Tomography Imaging” (16/415,430) pending, and the patent “Systems and Methods for Utilizing Synthetic Medical Images Generated Using a Neural Network” (62/962,641) pending. In addition M. Olender reports grants from MathWorks while conducting the study; and E.R. Edelman reports grants from the U.S. National Institutes of Health while conducting the study; grants from Abiomed, grants from Edwards LifeSciences, grants from Boston Scientific, grants from Medtronic, grants from Autus Medical, other from Biodevek, other from Panther Therapeutics, personal fees from Abbvie, outside the submitted work.
REFERENCES
1. Douglas PS, Pontone G, Hlatky MA, et al. Clinical outcomes of fractional flow reserve by computed tomographic angiography-guided diagnostic strategies vs. usual care in patients with suspected coronary artery disease:The prospective longitudinal trial of FFRCT:Outcome and resource impacts stud. Eur Heart J. 2015;36:3359-3367.
2. Athanasiou LS, Nezami FR, Edelman ER. Computational Cardiology. IEEE J Biomed Health Inform. 2019;23:4-11.
3. Stary HC, Chandler AB, Dinsmore RE, et al. A Definition of Advanced Types of Atherosclerotic Lesions and a Histological Classification of Atherosclerosis. Circulation. 1995;92:1355-1374.
4. Gray RA, Pathmanathan P. Patient-Specific Cardiovascular Computational Modeling:Diversity of Personalization and Challenges. J Cardiovasc Transl Res. 2018;11:80-88.
5. Corral-Acero J, Margara F, Marciniak M, et al. The 'Digital Twin'to enable the vision of precision cardiology. Eur Heart J. 2020;41:4556-4564.
6. Sakellarios AI, Pelosi G, Fotiadis DI, et al. Predictive Models of Coronary Artery Disease Based on Computational Modeling:The SMARTool System. Annu Int Conf IEEE Eng Med Biol Soc. 2019;2019:7002-7005.
* Corresponding author: 77 Massachusetts Avenue, Cambridge, MA 02139, United States.
E-mail address: molender@mit.edu (M. Olender).
Subcategories
Editorials
Percutaneous coronary intervention of the left main in the elderly: a reasonable option
Department of Cardiology and Angiology, University Heart Center Freiburg · Bad Krozingen, Medical Center – University of Freiburg, Faculty of Medicine, University of Freiburg, Freiburg, Germany
Original articles
Editorials
Are we ripe for preventive percutaneous coronary interventions?
aDepartment of Cardiology, McGill University Health Center, Montreal, Quebec, Canada
bDepartment of Structural Heart Disease, Silesian Medical University, Katowice, Poland
Original articles
Debate
Debate: Preventive coronary intervention for vulnerable plaque
The clinical cardiologist’s approach
Servicio de Cardiología, Hospital Universitario de Jaén, Jaén, Spain
The interventional cardiologist’s approach
Departamento de Cardiología, Hospital Universitari de Bellvitge, Institut d’Investigació Biomèdica de Bellvitge (IDIBELL), Universitat de Barcelona, L’Hospitalet de Llobregat, Barcelona, Spain