Available online: 09/04/2019
Editorial
REC Interv Cardiol. 2020;2:310-312
The future of interventional cardiology
El futuro de la cardiología intervencionista
Emory University School of Medicine, Atlanta, Georgia, United States
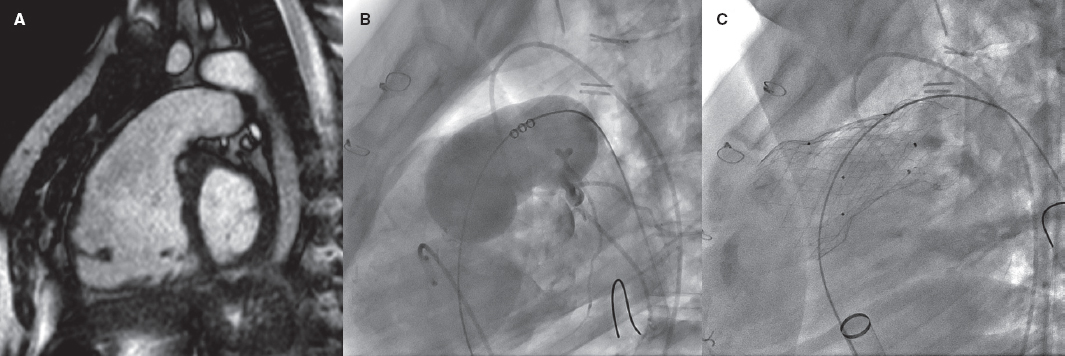
INTRODUCTION
Right ventricular outflow tract (RVOT) disease is a common finding in children and adults with congenital heart disease and often occurs as a sequel of previous surgery. Over the past 2 decades, percutaneous pulmonary valve implantation has become more widely used and is recommended by current clinical practice guidelines1 as the preferred option for patients with previous conduits or bioprostheses.
However, many patients have native or patched tracts (hereafter referred to as native RVOTs) with pulmonary regurgitation as the predominant lesion. In these patients, percutaneous valve placement is more complex due to the RVOT anatomy, its dynamic behavior, larger pulmonary annulus size, and lack of a proper landing zone for the valve. Because of the differences in underlying heart diseases, previous surgical repairs, and various pulmonary artery configurations, RVOT morphology varies widely but can be categorized into 5 subtypes2 (figure 1).
Figure 1. Five types of native RVOT anatomy: I - pyramidal; II - cylindrical or tubular; III - inverted pyramidal; IV - central enlargement; V - central narrowing. (Reproduced from Schievano et al.2 with permission from the author.)
Repaired tetralogy of Fallot serves as the paradigm, and in these cases, surgery remains the standard of care. However, the development of percutaneous procedures has enabled a larger number of patients with these substrates to be eligible for percutaneous treatment (figure 2).
Figure 2. Self-expanding pulmonary valve implantation in an aneurysmal right ventricular outflow tract (RVOT). A: severely dilated RVOT on magnetic resonance imaging, with interventricular septal flattening. B: balloon sizing test in the RVOT with simultaneous injection into the left main coronary artery. C: successful venus-P valve (MedTech, China) implantation in the RVOT.
Two different models of balloon-expandable valves have been authorized to treat dysfunctional bioprostheses and conduits: the Melody (Medtronic, United States) and the SAPIEN valves (XT model, Edwards Lifesciences, United States). Although they have not yet been authorized for implantation in native RVOTs, both (along with the SAPIEN S3) have been used off-label in this setting.
To address the specific characteristics of native RVOTs, several models of self-expanding valves have been developed, such as the Venus-P (Venus MedTech, China, with CE marking for use in Europe since 2022), PULSTA (TaeWoong Medical, South Korea), and Harmony valves (Medtronic, United States, with prior FDA approval). The Alterra valve (Edwards Lifesciences) has also been used. This valve serves as a self-expanding prestent onto which a SAPIEN valve is later implanted.
The characteristics of each of these devices have already been described in detail in a previous issue of REC: Interventional Cardiology.3
RESULTS OF PERCUTANEOUS VALVES IN THE NATIVE RIGHT VENTRICULAR OUTFLOW TRACT
More information has gradually become available on the favorable results and durability of percutaneous valves. The largest multicenter registry to date,4 with 2476 patients (82% implanted with the Melody valve and 18% with the SAPIEN device, including 16% with native RVOTs), reported an 8-year survival rate of 91.1% after implantation, and a reintervention rate of 25.1%, which is similar to the rates reported in some surgical series.5 Nonrandomized comparative studies6 and a recent meta-analysis7 also found similar reintervention rates. Some series report higher rates in patients implanted with the Melody compared with the SAPIEN valve,8,9 although the 2 groups were not directly comparable, with reintervention-free survival rates in patients with SAPIEN being similar to those reported in patients with surgical valves.8
The SAPIEN device can be implanted with or without prestenting, depending on the patient’s characteristics, with good outcomes. The largest trial published to date included 774 patients implanted with the XT and S3 models10, 51% of whom had native RVOTs (table 1).
Table 1. Summary of some of the main trials of patients with native right ventricular outflow tract
Author and year | Patients with native RVOT/Total patients | Valve type | Follow-up | Implant success | Other results | Complications |
---|---|---|---|---|---|---|
Malekzadeh-Milani et al.23, 2014 | 34/34 | Melody | 2.6 years | 100% | Paravalvular leak in 2 patients during follow-up | 3 acute complications (9%): 1 hemoptysis, 1 RVOT obstruction, 1 stent embolization |
Meadows et al.18, 2014 | 31/31 | Melody | 15 months (1 month- 3.8 years) | 100% | No mortality or valve regurgitation | Stent fractures (32%) associated with a higher rate of stenosis. 3 cases of endocarditis. Reintervention in 3 patients |
Garay et al.13, 2017 | 10/10 | Venus P | 12 months (4 to 21) | 100% | Normally functioning valve, no stent fractures, right ventricular remodeling, and NYHA functional class improvement | None |
Martin et al.11, 2018 | 132/132 | Melody | No follow-up | Complete cohort of 229 patients, but only 58% implanted. Good immediate hemodynamic outcomes | Complication rate of 4% (mostly due to stent migration) | |
Morgan et al.24, 2019 | 41/57 | SAPIEN (S3, XT) | 5.3 months (1 to 26) | 100% | No prestenting. Normally functioning valve at follow-up. No mortality | 1 aortic compression, 2 tricuspid valve injury, 1 valve regurgitation |
Shahanavaz et al.10, 2020 | 397/774 | SAPIEN S3 (78%) XT (22%) | 12 months (n = 349) | 97.4% | Normally functioning valve: 91.5% | Adverse events: 10%. Emergency surgery: 14 patients (1.8%). Tricuspid injury: 3% |
Goldstein et al.19, 2020 | 143/530 | Melody (88%) SAPIEN (22%) | 1 year | 98% | Normally functioning valve: 98% | 1 death. Reintervention rate of 13.3% (mostly unrelated to the valve) |
Lee et al.12, 2021 | 25/25 | PULSTA | 33 (± 14) months | 100% | Zero cases of valve dysfunction | No significant adverse events |
Gillespie et al.16, 2021 | 21/21 | Harmony | 5 years | 100% | Implantation in all but 1 patient due to pulmonary hypertension. Normally functioning valve in nonreoperated patients | Valve explantation in 2 patients, 1 death 3 years after implantation, 2 reinterventions (valve-in-valve) |
Morgan et al.25, 2021 | 38/38 | Venus | 27 months | 97.4% | Normally functioning valve at follow-up | Migration: 2 cases (surgery in 1) |
Houeijeh et al.9, 2023 | 99/214 | SAPIEN XT/S3 (85%) Melody (15%) | 2.8 years (3 months-11.4 years) | Only cases with successful implantation included | Reintervention-free survival at 5 to 10 years: 78.1% to 50.4% (Melody) and 94.3% to 82.2% (SAPIEN) | Severe complications: 2.3%, 1 valve-related death. Endocarditis 5.5/100 patient-years (Melody) and 0.2/100 patient-years (SAPIEN) |
Álvarez et al.14, 2023 | 8/8 | Venus | No follow-up | 100% | Normally functioning valve in all | No significant adverse events |
Lin et al.15, 2023 | 53/53 | Venus (28%), PULSTA (72%) | 27.5 months | 98.1% | No valve regurgitation at 12 months | 1 embolization, 1 endocarditis |
NYHA, New York Heart Association; RVOT, right ventricular outflow tract. In studies that are not specific to native RVOT, the results and complications refer to the overall cohort, as the results of native, or non-native RVOTs are often not detailed independently. Some centers participated in > 1 study, which allowed the same patient to be included in multiple publications. The most recent series with larger numbers of patients have been prioritized. |
In a study of patients with native RVOTs that included 229 candidates for the Melody valve, the device was finally implanted in 132 patients (58%).11 The most common reason for avoiding implantation was a prohibitively large RVOT, followed by coronary or aortic root compression. The immediate outcomes of patients with successful implantation were good. However, the low implantation rate demonstrates the limitation of treating native RVOTs with these valves.
Self-expanding valves fill this gap by allowing treatment of larger RVOTs, as they adapt to the anatomy of the RVOT and provide more stable attachment. The series published to date indicate a very high implantation success rate—close to 100%—with good short- and mid-term outcomes and few complications.12-16 (table 1 illustrates a selection of series representative of patients with native RVOTs).
Drawing comparisons between the results of surgical and percutaneous pulmonary valves is challenging because the types of patients and the anatomies treated are very different. Overall, patients undergoing percutaneous valve implantation are at higher risk and often have bioprostheses or small conduits that require smaller percutaneous valves, which is associated with a higher residual gradient, leading to a greater need for reintervention,4,8,9 and a higher rate of endocarditis. This aspect has traditionally led to less favorable results with the Melody series (with a maximum diameter of 22 mm), with durability being one of the issues initially identified, although more recent series have shown positive results. Only indirect comparisons can be drawn with valve procedures in the native RVOT.
PROCEDURAL PLANNING
Multimodal imaging modalities, especially magnetic resonance imaging and computed tomography, are crucial for patient selection and procedural planning. Key aspects include measurement of the RVOT and the pulmonary annulus, and assessment of both the RVOT and the coronary anatomy. Recently, computed tomography guidelines for RVOT assessment17 have been published with detailed information on the measurements that should be taken, and the cardiac cycle phase.
POTENTIAL PROBLEMS
Valve or stent migration or embolization is a potential complication in patients with large RVOTs due to the lack of an adequate landing zone, with incidence rates between 0% and 4.5%,11,10,18 and consequently proper valve sizing is of paramount importance.
Stent fractures can lead to loss of integrity and contribute to prosthetic valve dysfunction. Nonetheless, the implications of the occasional finding of an isolated strut fracture remain unclear.
Tricuspid valve injury has been reported in 3% to 6% of patients.10 However, this rate has dropped significantly with the use of DrySeal introducer sheaths (W.L. Gore & Associates, United States).10
Coronary compression is a rare complication nowadays, because coronary angiography is simultaneous and systematically performed during RVOT balloon inflation testing. However, it can be a reason for not performing percutaneous implantation in nearly 3% of patients.11
A higher incidence of infective endocarditis has been reported, especially after Melody valve implantation,6,8,9 with a higher risk when smaller valves are implanted and there is a greater residual gradient. The risk involved with other types of device such as the SAPIEN—whether because of its different composition (bovine jugular vein graft in the Melody compared with bovine pericardium in the SAPIEN) or because of its larger size—is much lower9 and seems comparable to that of surgical series.
Self-expanding valves are larger, and the proximal end often remains inside the RVOT, which could increase the risk of ventricular arrhythmias. The incidence of nonsustained ventricular tachycardia varies widely (between 0.6% and 40%11,19,20), although it is usually a transient phenomenon during the early postimplantation phase, and its long-term implications remain unclear. Of note, when comparing surgical with percutaneous pulmonary valve replacement, the early incidence of arrhythmias was lower in the latter.21 A potential caveat is that catheter access to the arrhythmic substrate can be limited after valve implantation.
The presence of the valve metal mesh with or without previous stents inside the RVOT can pose additional challenges for the surgeon if surgical valve replacement is subsequently required. This is a relative problem, because surgical pulmonary valve replacement also increases the risk of future reinterventions related to resternotomy.
BENEFITS OF PERCUTANEOUS VALVE IMPLANTATION
The possibility of performing percutaneous pulmonary valve implantation offers clear advantages: the procedure is much less invasive, length of stay is shorter,8 recovery is faster, the mortality rate is very low (from 0.2% to 0.8%),19 and the cost-effectiveness ratio is more favorable.22 In patients at high surgical risk, it might be the only available treatment option. The path followed by its “left-sided relatives”—transcatheter heart valves in the aortic position—illustrates that the threshold for the use of percutaneous techniques is decreasing as more experience is gained and technology becomes further refined. In cases of intermediate or low risk, percutaneous valve implantation may delay or avoid the need for surgery in patients who often require multiple interventions during their lifetime. This concept of avoiding sternotomies is relevant due to the added risk of further surgeries and for patients who are candidates for heart transplants. The decision on the best approach to valve implantation in each patient should be made by a multidisciplinary heart team, including health professionals experienced in these types of heart disease.
CONCLUSIONS
Percutaneous pulmonary valve implantation is particularly challenging in patients with native RVOTs. Nonetheless, it is a feasible option that is being used with a high success rate and few complications. However, appropriate candidate selection is essential. Several models of self-expanding valves have been specifically developed for this purpose, with good short- and mid-term results, allowing the treatment of patients with large RVOTs that were previously not amenable to balloon-expandable devices. The latest information suggests that the durability of percutaneous valves may be comparable to that of surgical bioprostheses, although long-term data are lacking, especially with the latest models. Although more studies and follow-up are necessary, percutaneous techniques are already an option for many patients and will likely become an alternative to surgical treatment in the near future.
FUNDING
None declared.
CONFLICTS OF INTEREST
None declared.
REFERENCES
1. Baumgartner H, De Baker J, Babu-Narayan S, et al. 2020 ESC Guidelines for the management of adult congenital heart disease. The Task Force for the management of adult congenital heart disease of the European Society of Cardiology (ESC). Eur Heart J. 2021;42:563-645.
2. Schievano S, Coats L, Migliavacca F, et al. Variations in Right Ventricular Outflow Tract Morphology Following Repair of Congenital Heart Disease:Implications for Percutaneous Pulmonary Valve Implantation. J Cardiovasc Magn Reson. 2007;9:687-695.
3. Gutiérrez-Larraya Aguado F, Pardeiro CA, Domingo EJB. Percutaneous treatment of pulmonary valve and arteries for the management of congenital heart disease. REC Interv Cardiol. 2021;3:119-128.
4. McElhinney DB, Zhang Y, Levi DS, et al. Reintervention and Survival After Transcatheter Pulmonary Valve Replacement. J Am Coll Cardiol. 2022;79:18-32.
5. Buber J, Egidy G, Huang A, et al. Durability of large diameter right ventricular out fl ow tract conduits in adults with congenital heart disease. Int J Cardiol. 2023;175:455-463.
6. Georgiev S, Ewert P, Eicken A, et al. Munich Comparative Study:Prospective Long-Term Outcome of the Transcatheter Melody Valve Versus Surgical Pulmonary Bioprosthesis with up to 12 Years of Follow-Up. Circ Cardiovasc Interv. 2020;13:1-7.
7. Ribeiro JM, Gonc L, Costa M. Transcatheter Versus Surgical Pulmonary Valve Replacement:A Systemic Review. Ann Thorac Surg. 2020;110:1751-1761.
8. Hribernik I, Thomson J, Ho A, et al. Comparative analysis of surgical and percutaneous pulmonary valve implants over a 20-year period. Eur J Cardiothoracic Surg. 2022;61:572-579.
9. Houeijeh A, Batteux C, Karsenty C, et al. Long-term outcomes of transcatheter pulmonary valve implantation with melody and SAPIEN valves. Int J Cardiol. 2023;370:156-166.
10. Shahanavaz S, Zahn EM, Levi DS, et al. Transcatheter Pulmonary Valve Replacement With the SAPIEN Prosthesis. J Am Coll Cardiol. 2020;76:2847-2858.
11. Martin MH, Meadows J, McElhinney DB, et al. Safety and Feasibility of Melody Transcatheter Pulmonary Valve Replacement in the Native Right Ventricular Outflow Tract:A Multicenter Pediatric Heart Network Scholar Study. JACC Cardiovasc Interv. 2018;11:1642-1650.
12. Lee S, Kim S, Kim Y. Mid-term outcomes of the Pulsta transcatheter pulmonary valve for the native right ventricular outflow tract. Catheter Cardiovasc Interv. 2021;98:E724-E732.
13. Garay F, Pan X, Zhang YJ, Wang C, Springmuller D. Early experience with the Venus p-valve for percutaneous pulmonary valve implantation in native outflow tract. Netherlands Hear J. 2017;25:76-81.
14. Álvarez-Fuente M, Toledano M, Hernández I, et al. Initial experience with the new percutaneous pulmonary self-expandable Venus P-valve. REC Interv Cardiol. 2023;5:263-269.
15. Lin MT, Chen CA, Chen SJ, et al. Self-Expanding Pulmonary Valves in 53 Patients With Native Repaired Right Ventricular Outflow Tracts. Can J Cardiol. 2023;39:997-1006.
16. Gillespie MJ, Bergersen L, Benson LN, Weng S, Cheatham JP. 5-Year Outcomes From the Harmony Native Outflow Tract Early Feasibility Study. JACC Cardiovasc Interv. 2021;14:816-817.
17. Han BK, Garcia S, Aboulhosn J, et al. Technical recommendations for computed tomography guidance of intervention in the right ventricular outflow tract:Native RVOT conduits and bioprosthetic valves:A white paper of the Society of Cardiovascular Computed Tomography (SCCT), Congenital Heart Surgeons'Society (CHSS), and Society for Cardiovascular Angiography &Interventions (SCAI). J Cardiovasc Comput Tomogr. 2023;18(2024):75-99.
18. Meadows JJ, Moore PM, Berman DP, et al. Congenital Heart Disease Use and Performance of the Melody Transcatheter Pulmonary Valve in Native and Postsurgical, Nonconduit Right Ventricular Outflow Tracts. Circ Cardiovasc Interv. 2014;7:374-380.
19. Goldstein BH, Bergersen L, Armstrong AK, et al. Adverse Events, Radiation Exposure, and Reinterventions Following Transcatheter Pulmonary Valve Replacement. J Am Coll Cardiol. 2020;75:363-376.
20. Taylor A, Yang J, Dubin A, et al. Ventricular arrhythmias following transcatheter pulmonary valve replacement with the harmony TPV25 device. Catheter Cardiovasc Interv. 2022;100:766-773.
21. Wadia SK, Lluri G, Aboulhosn JA, et al. Ventricular arrhythmia burden after transcatheter versus surgical pulmonary valve replacement. Heart. 2018;104:1791-1796.
22. Vergales JE, Wanchek T, Novicoff W, Kron IL, Lim DS. Cost-Analysis of Percutaneous Pulmonary Valve Implantation Compared to Surgical Pulmonary Valve Replacement. Catheter Cardiovasc Interv. 2013;82:1147-1153.
23. Malekzadeh-Milani S, Ladouceur M, Cohen S, Iserin L, Boudjemline Y. Results of transcatheter pulmonary valvulation in native or patched right ventricular outflow tracts. Arch Cardiovasc Dis. 2014;107:592-598.
24. Morgan GJ, Sadeghi S, Salem MM, et al. SAPIEN valve for percutaneous transcatheter pulmonary valve replacement without “pre-stenting“:A multi-institutional experience. Catheter Cardiovasc Interv. 2019;93:324-329.
25. Morgan G, Prachasilchai P, Promphan W, et al. Medium-term results of percutaneous pulmonary valve implantation using the Venus P-valve:international experience. EuroIntervention. 2019;14:1363-1370.
* Corresponding author.
E-mail address: merascolunga@gmail.com (P. Merás Colunga).
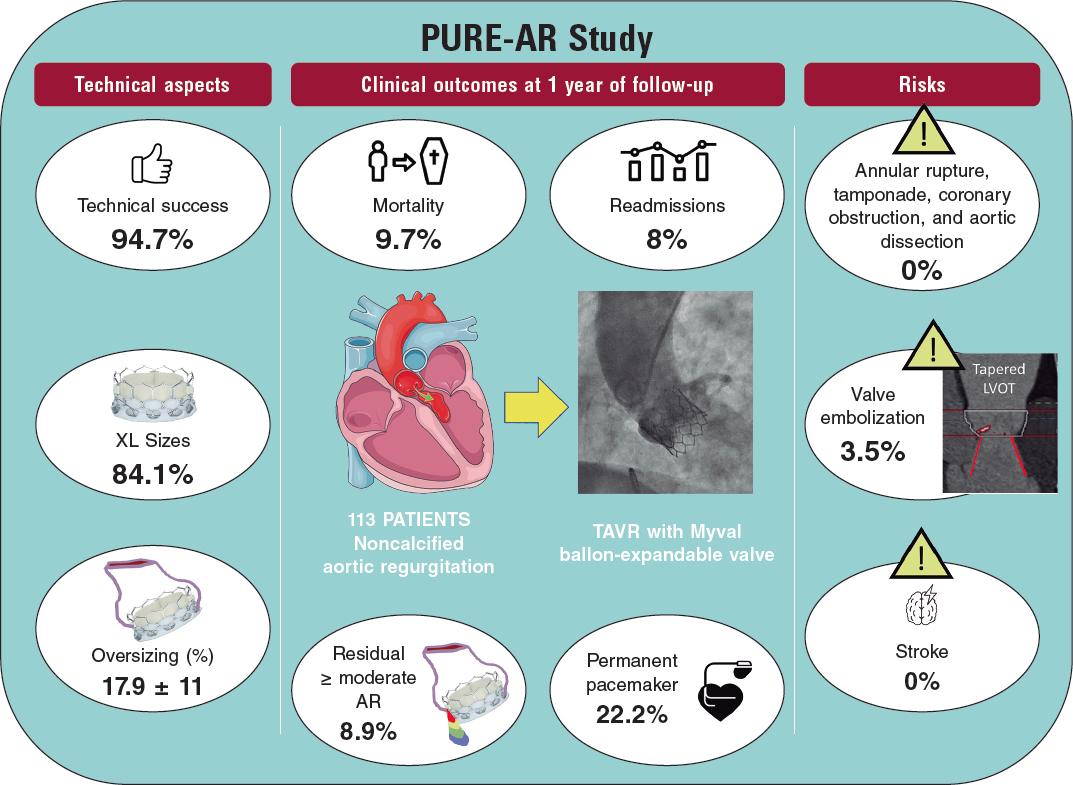
INTRODUCTION
Transcatheter aortic valve implantation (TAVI) is a well-established procedure for severe symptomatic aortic stenosis. Previously, this procedure was used exclusively to treat inoperable and high-risk patients but is now a common approach for intermediate and low-risk populations. In parallel, there has been growing global experience in several off-label scenarios, as in bicuspid, valve-in-valve, and noncalcified aortic regurgitation (NCAR).1
AORTIC REGURGITATION
Due to abnormalities in the aortic leaflets or their supporting structures (ie, aortic root and annulus), or both, aortic regurgitation (AR) causes diastolic reflux of blood from the aorta into the left ventricle (LV). This leads to LV volume overload and dilatation, allowing the ejection of a larger stroke volume. However, over time, it results in a decline in systolic function causing symptoms conferring a poor prognosis.2 Current European and American guidelines recommend surgical intervention when significant AR is accompanied by symptoms, reduced LV ejection fraction, or severe LV dilatation.3-4 Although moderate or severe AR affects around 2.2% of the population aged 70 years or older, up to 30% of these individuals are deemed inoperable due to advanced age or comorbidities, with percutaneous options—including dedicated and nondedicated devices—gaining increased interest due to the absence of safe surgical alternatives.5
CHALLENGES OF TAVI IN NCAR
Specific challenges for transcatheter devices are due to the characteristics of patients with NCAR, including larger aortic annular dimensions, aortic root dilatation, insufficient annular calcification for anchoring, and larger stroke volume with backflow to the LV causing a “suction effect”. All of these factors create difficulties in selecting the appropriate device and positioning and deploying it correctly, and consequently increase the risk of embolization or malpositioning of the prosthesis, requiring a second valve implantation. Ultimately, these challenges are associated with increased mortality. In particular, the primary concern is valve embolization. To reduce the risk of this complication, prosthesis oversizing is routinely performed, but the exact degree of oversizing with each device has not been standardized. Furthermore, oversizing is associated with a high rate of conduction system disturbances and may carry a higher risk of annular rupture.
DEVICES AND EVIDENCE
There are 2 dedicated-devices for NCAR: The Trilogy system (JenaValve Technology Inc, California, United States) and the J-Valve (JC Medical Inc, California, United States). The most recent experience with these dedicated devices has been reported by Adam et al.6 and García et al.7 The Trilogy system was associated with a 30-day mortality rate of 1.7% (vs 3.7% with J-Valve) and both had 0% ≥ moderate residual AR. However, 7.4% required conversion to surgery with the J-Valve, leading to some technical changes in the technology. Of note, the pacemaker rate with both systems was above 10% (19.6% and 13%, respectively). These promising dedicated technologies still have certain shortcomings, the main one being the lack of sizes covering large annuli; indeed, for 10% oversizing, the proportion of AR patients whose aortic structures were above the recommended indications was up to ~50%.8
The first-in-human compassionate use of SAPIEN (Edwards Lifesciences, California, United States) was reported in 2012,9 followed by multiple case series and registries reporting the feasibility of TAVI in NCAR with new-generation nondedicated devices. The most recent summary of the experience was reported in a meta-analysis by Takagi et al.,10 and the pooled analysis at 30 days demonstrated 80.4% device success, 9.5% all-cause mortality, 7.4% ≥ moderate residual AR, and a pacemaker rate of 11.6%. Although these results were promising and significantly better than with early-generation devices in all aspects, there was still a substantial gap to achieve similar outcomes to TAVI in aortic stenosis and, indeed, the rate of valve embolization was high (above 9% with all the technologies, even the dedicated technologies).10
More recently, the new Myval balloon-expandable valve (Meril Lifesciences Ltd, Vapi, India) has demonstrated better outcomes than those reported by Takagi et al. likely due to the availability of extra-large sizes (30.5 and 32 mm), allowing a greater degree of oversizing and covering annuli up to 100.5 mm perimeter and 840 mm2 area at its nominal volume, increasing the proportion of inoperable patients who can be treated percutaneously. Because of the high procedural success rate (94.7%), and the absence of severe anatomical complications (ie, annular rupture, aortic dissection, or coronary obstruction), the Myval balloon-expandable valve is a promising new approach to this condition until dedicated devices for these annular sizes become available. Nevertheless, prosthesis embolization occurs in 3.5% of patients, leaving room for improvement; according to this research, the interplay of the aortic annulus and the LV outflow tract could help to predict the risk of valve embolization, suggesting that, in borderline annular sizes, when 20% oversizing cannot be achieved and adverse (tapered) morphology is detected, the intervention should be avoided or might be carried out under mechanical circulatory support (figure 1).11
Figure 1. Anatomical predictor of valve embolization during transcatheter aortic valve implantation for noncalcified aortic regurgitation. LVOT, left ventricular outflow tract.
Poletti et al.12 recently compared latest-iteration nondedicated devices and suggested that Myval might provide the best outcomes compared with other devices; indeed, the subanalysis (not published yet) comparing the 2 balloon-expandable platforms suggested that, despite being used in patients with significantly smaller aortic annuli, SAPIEN-3 had a lower device success rate (72% vs 90%) and a higher rate of prosthesis migration/embolization (SAPIEN 17% vs MyVal 5%).12 A summary of the PURE-AR study11 with the Myval device is shown in figure 2. The current evidence on different devices is summarized in table 1.
Figure 2. Main outcomes reported in the Myval registry for treatment of noncalcified aortic annuli with large annuli. AR, aortic regurgitation; LVOT, left ventricular outflow tract; TAVR, transcatheter aortic valve regurgitation.
Table 1. Comparison of outcomes between the international registries
Registry | No. of patients | Type of device | Device success | All-cause mortality | ≥ Moderate residual aortic regurgitation | Permanent pacemaker implantation rate |
---|---|---|---|---|---|---|
Yoon et al.13 (2017) | 331 patients with NCAR and FSHV | Nondedicated devices | Overall: 74.3% | Overall: 10.9% | Overall: 9.6% | Overall: 18.2% |
Early-generation devices (CoreValve, SAPIEN XT) | Early-generation devices: 61.3% | Early-generation devices: 13.4% | Early-generation devices: 18.8% | Early-generation devices: 17.5% | ||
New-generation devices (Evolut R, JenaValve, Engager, Portico, ACURATE, Lotus, Direct Flow, SAPIEN 3) | New-generation devices: 81.1% | New-generation devices: 9.4% | New-generation devices: 4.2% | New-generation devices: 18.6% | ||
De Backer et al.14 (2018) | 254 patients with NCAR | Nondedicated devices Early-generation devices (CoreValve, SAPIEN XT) | Early-generation devices: 47% | Early-generation devices: 17% | Early-generation THV’s: 26% | Not reported |
New-generation devices (Evolut R, JenaValve, Engager, Portico, ACURATE, Lotus, Direct Flow, SAPIEN 3) | Newer-generation devices: 82% | Newer-generation devices: 8% | Newer-generation THV’s: 5% | |||
Sawaya et al.15 (2018) | 146 patients with NCAR and failing surgical heart valves (FSHV) | Early-generation devices (CoreValve SAPIEN XT) | NCAR: 72% | NCAR: 13% | NCAR: 13% | NCAR: 18% |
New-generation devices (Evolut R, JenaValve, Lotus, Direct Flow, SAPIEN 3) | FSHV: 71% | FSHV: 6% | FSHV: 6% | FSHV: 5% | ||
Early-generation devices: 54% | Early-generation devices: 22% | Early-generation devices: 27% | ||||
New-generation devices: 85% | New-generation devices: 8% | New-generation devices: 3% | ||||
Sánchez-Luna et al.11 2023 | 113 patients with NCAR | New-generation nondedicated device: Myval | 94.7% | 9.7% | 8.9% | 22.2% |
Poletti et al.12 2023 | 201 patients with NCAR | New-generation devices: SEV (Evolut R/Pro, ACURATE Neo/Neo2, Jena Valve, Navitor/Portico) | Overall: 76.1% | Overall: 5% | Overall: 9.5% | Overall: 22.3% |
SEV: 75.8% | SEV: 5.3% | SEV: 9.2% | SEV: 22.6% | |||
BEV (SAPIEN, Myval) | BEV: 76.8% | BEV: 4.4% | BEV: 10.1% | BEV: 21.8% | ||
Adam et al.6 (2023) | 58 patients with NCAR | Dedicated device: Trilogy system (JenaValve) | 98% | 1.7% | 0% | 19.6% |
Garcia S et al.7 (2023) | 27 patients with NCAR | Dedicated device: J-Valve | 81% | 3.7% | 0% | 13% |
BEB, balloon-expandable valve; FSHV, failing surgical heart valve; NCAR, noncalcified aortic regurgitation; SEV, self-expandable valve; THV, transcatheter heart valve. Medtronic, United States: CoreValve, Engager, and Evolut R. Edwards Lifesciences, United States: SAPIEN XT, SAPIEN 3. Abbott Vascular, United States: Navitor/Portico. Meril Life, India: Myval. Boston Scientific, United States: ACURATE Neo/Neo2, Lotus. Trilogy, Germany: JenaValve. Direct Flow Medical, United States: Direct Flow. JC Medical, United States: J-Valve. |
In conclusion, although the surgical approach remains the standard-of-care in NCAR patients, the outcomes of new-generation dedicated and nondedicated TAVI devices are rapidly improving. The main caveats include the lack of large-sized dedicated devices and the risk of valve embolization with nondedicated devices, although this risk can be minimized by extra-large sizes and morphological analysis of the LV outflow tract. The rate of conduction disturbances is still higher than for TAVI in aortic stenosis and its decrease will require specific technology and strategies, given its potentially negative impact in LV remodelling. Clinical trials comparing surgery and TAVI in this setting for high-risk patients are an urgent need.
FUNDING
None.
AUTHORS’ CONTRIBUTIONS
I.J. Amat-Santos and J.P. Sánchez-Luna equally contributed in the design, data gathering, analysis, and final approval of the manuscript.
CONFLICTS OF INTEREST
I.J. Amat-Santos is proctor for Boston Scientific, Medtronic, and Meril Life. There are no other potential conflicts of interest related to this work.
REFERENCES
1. Santos-Martínez S, Amat-Santos IJ. New Challenging Scenarios in Transcatheter Aortic Valve Implantation: Valve-in-valve, Bicuspid and Native Aortic Regurgitation. Eur Cardiol. 2021;16:e29.
2. Maurer G. Aortic regurgitation. Heart. 2006;92:994-1000.
3. Vahanian A, Beyersdorf F, Praz F, et al. 2021 ESC/EACTS Guidelines for the management of valvular heart disease. EuroIntervention. 2022;17:e1126-e1196.
4. Otto CM, Nishimura RA, Bonow RO, et al. 2020 ACC/AHA Guideline for the Management of Patients With Valvular Heart Disease: Executive Summary: A Report of the American College of Cardiology/American Heart Association Joint Committee on Clinical Practice Guidelines. Circulation. 2021;143:e35-e71.
5. Singh JP, Evans JC, Levy D, et al. Prevalence and clinical determinants of mitral, tricuspid, and aortic regurgitation (the Framingham Heart Study). Am J Cardiol. 1999;83:897-902.
6. Adam M, Tamm AR, Wienemann H, et al. Transcatheter Aortic Valve Replacement for Isolated Aortic Regurgitation Using a New Self-Expanding TAVR System. JACC Cardiovasc Interv. 2023;16:1965-1973.
7. Garcia S, Ye J, Webb J, et al. Transcatheter treatment of native aortic valve regurgitation: The North American Experience with a Novel Device. JACC Cardiovasc Interv. 2023;16:1953-1960.
8. Chen Y, Zhao J, Liu Q, et al. Computed tomography anatomical characteristics based on transcatheter aortic valve replacement in aortic regurgitation. Int J Cardiovasc Imaging. 2023;39:2063–2071.
9. D'Ancona G, Pasic M, Buz, S, et al. TAVI for pure aortic valve insufficiency in a patient with a left ventricular assist device. Ann Thorac Surg. 2012;93:e89-e91.
10. Takagi H, Hari Y, Kawai N, et al. Meta-analysis and meta-regression of transcatheter aortic valve implantation for pure native aortic regurgitation. Heart Lung Circ. 2020;29:729-741.
11. Sánchez-Luna JP, Martin P, Dager AE, et al. Clinical outcomes of TAVI with the Myval balloon-expandable valve for non-calcified aortic regurgitation. EuroIntervention. 2023;19:580-588.
12. Poletti E, De Backer O, Scotti A, et al. Transcatheter Aortic Valve Replacement for Pure Native Aortic Valve Regurgitation: The PANTHEON International Project. JACC Cardiovasc Interv. 2023;16:1974-1985
13. Yoon SH, Schmidt T, Bleiziffer S, et al. Transcatheter Aortic Valve Replacement in Pure Native Aortic Valve Regurgitation. J Am Coll Cardiol. 2017;70:2752-2763.
14. De Backer O, Pilgrim T, Simonato M, et al. Usefulness of Transcatheter Aortic Valve Implantation for Treatment of Pure Native Aortic Valve Regurgitation. Am J Cardiol. 2018;122:1028-1035.
15. Sawaya FJ, Deutsch MA, Seiffert M, et al. Safety and Efficacy of Transcatheter Aortic Valve Replacement in the Treatment of Pure Aortic Regurgitation in Native Valves and Failing Surgical Bioprostheses: Results From an International Registry Study. JACC Cardiovasc Interv. 2017;10:1048-1056.
Transcatheter aortic valve implantation (TAVI) is an established treatment for elderly patients with severe symptomatic aortic stenosis. Currently, most procedures are performed through transfemoral transcatheter aortic valve implantation (TF-TAVI) under conscious sedation via vascular access with safe and effective closure.1 Nonetheless, large-bore transcatheter arterial accesses are inherently associated with a higher risk of vascular and hemorrhagic complications.2
The use of vascular closure devices (VCDs) in endovascular procedures has grown exponentially. Their emergence has reduced time to hemostasis, facilitated early ambulation and discharge, and reduced hospitalization costs.2-4
However, failed VCDs are not an uncommon finding (1%-8%), and vascular and hemorrhagic complications—most commonly found at the access site—can sometimes determine procedural success or failure.5,6 Vascular complications significantly contribute to clinical outcomes in the short- and long-term, including length of stay, rehospitalizations, need for blood transfusions, and all-cause mortality.2,4 Therefore, successful arterial access and closure is critical in TAVI.2,7,8
CURRENT DEVICES
The most widely used VCDs for large-bore arteriotomy closure are the suture-mediated closure system, Perclose ProGlide system (Abbott Vascular, United States), and the most recent plug-based MANTA vascular closure device (Teleflex/Essential Medical, United States).
The failure mechanisms associated with the Perclose ProGlide device include suture-related malfunction, unsuccessful deployment, and incomplete vessel wall apposition. Potential errors associated with the MANTA device include failed deployment, intraluminal or subcutaneous deployment, collagen detachment, delivery handle-related arterial occlusion, and incomplete handle apposition.5,6,8
The first feasibility studies ever conducted on the MANTA device showed promising safety and efficacy. Afterwards, several registries have presented their findings that compare favorably with suture techniques.8-11
Two recent randomized clinical trials have compared the 2 techniques. The MASH trial included 210 patients and found no differences in its primary endpoint: puncture site-related major and minor complications (10% with the MANTA vs 4% with the ProGlide; P = .16).6 In contrast, in the CHOICE-CLOSURE trial of 516 patients, the MANTA device was associated with a higher rate of puncture site-related major and minor vascular complications (19.4% vs 12% with ProGlide; P = .029), and a similar incidence of puncture-site bleeding (11.6% vs 7.4%; P = .133).12
In an article published in REC: Interventional Cardiology, Martinho et al.13 present an interesting single-center observational study on the largest real-world experience with the MANTA device. The aim of the study was to evaluate the safety and efficacy profile of the device in a consecutive unselected cohort of patients referred for TF-TAVI. The registry included 245 patients from March 2020 through February 2022. The participants’ median age was 81 years, 52.7% were women, and the median EuroSCORE II was 3.15%. The 30-day TF-TAVI puncture site-related vascular complications were studied according to the Valve Academic Research Consortium (VARC) criteria. Regarding the primary outcome measure of the study—efficacy according to the VARC-3 criteria—successful puncture-site closure was achieved in 92.2% of participants. There were no major vascular or hemorrhagic complications, and only 8.6% experienced minor vascular complications according to the VARC-3 criteria (secondary safety outcome measure). The main predictors of device failure were a minimum femoral artery diameter and, consequently, a higher sheath-to-femoral artery diameter ratio, and the presence of more tortuous and calcified arterial accesses.13
Compared with the most recent clinical trials, the study by Martinho et al.13 found rates of device failure that were lower than those in the MASH trial (7.8% vs 20%), but higher than those reported by the CHOICE-CLOSURE trial (4.7%). According to the authors, some of these differences may be attributed to the varying and inconsistent definitions of device failure across the studies.6,12
According to researchers, the study limitations include its single-center retrospective design, the absence of comparisons with other closure devices, and the lack of postoperative ultrasound scans for some of the participants (which were mandatory in the CHOICE-CLOSURE trial, potentially contributing to a higher detection rate of access site-related complications).12 We should also mention that the registry included only patients considered eligible for closure with the MANTA device and excluded those with “unsuitable” anatomies.
To date, the available information, which includes numerous observational studies, 2 current randomized controlled trials, and more than 1 meta-analysis, suggests that vascular and hemorrhagic complications associated with collagen-based VCDs are quite similar to those of suture-based devices. No significant differences in the VCD failure rate have been reported, with identical 30-day all-cause mortality rates in the 2 groups.
CONCLUSIONS
The gradual reduction in the rate of vascular complications is the result of better preoperative assessment and technological support. Meticulous preoperative planning, with multidetector computed tomography for iliofemoral arterial axis study and ultrasound-guided access, should allow for an optimal approach to the access site and avoidance of incorrect cannulations.
Undoubtedly, cannulation of severely calcified arteries is associated with worse outcomes because of the difficulty involved in attaching the suture systems, and the higher risk of poor plug device apposition. Conversely, puncture sites without wall calcification should consistently yield better results, regardless of the type of VCD used.
Finally, regardless of the system used, angiographic or ultrasound confirmation after closure is always necessary to identify undetected VCD failures, vascular occlusions, or subcutaneous deployments, and expedite the implementation of corrective measures.5,13
In the search for the ideal VCD, several criteria have been proposed: successful deployment with immediate hemostasis in ≥ 98% of procedures, reduction of device-related major vascular complications to ≤ 1%, versatility to adapt to challenging anatomies, universal applicability with minimal exclusions, user-friendliness, easy access to the arterial site after closure, and the potential for early ambulation.5 Such a perfect closure device, however, is still far from being a reality, which is why VCD innovation must continue to minimize vascular complications and achieve such goals.
A less well studied yet undoubtedly pivotal variable to achieve favorable outcomes with any VCD is the operator’s experience, which is key to guaranteeing closure success and reducing VCD-related complications. However, measuring, quantifying, and analyzing this variable across different studies is a daunting task. Thus, although the perfect device may never become a reality, there will always be experienced operators who prefer certain types of VCDs.
With the current results, the decision to choose one VCD over another should be based on the patient’s anatomy and basically on the operator’s preferences and experience. An important consideration is that, to date, the MANTA device is more expensive than ProGlide, which, added to the substantially larger number of patients treated with TAVI, could pose a major obstacle to its selection in preference to suture-based systems.
In the meantime, we should consider innovations and technological advancements in the field of VCDs as welcome news for the interventional community. Soon, these advancements will likely position TF-TAVI as the technique of choice for most patients with symptomatic aortic stenosis.
FUNDING
None declared.
CONFLICTS OF INTEREST
None declared.
REFERENCES
1. Goldsweig AM, Secemsky EA. Vascular Access and Closure for Peripheral Arterial Intervention. Intervent Cardiol Clin. 2020;9:117-124.
2. Montalto C, Munafo` AR, Arzuffi L, et al. Large-bore arterial access closure after transcatheter aortic valve replacement:a systematic review and network meta-analysis. Eur Heart J. 2022;2:1-9.
3. Carroll JD, Mack MJ, Vemulapalli S, et al. STS-ACC TVT registry of transcatheter aortic valve replacement. J Am Coll Cardiol. 2020;76:2492-2516.
4. Bhogal S, Waksman R. Vascular Closure:the ABC's. Curr Cardiol Rep. 2022;24:355-364.
5. Abbott JD, Bavishi C. In Search of an Ideal Vascular Closure Device for Transcatheter Aortic Valve Replacement. JACC Cardiovasc Interv. 2021;14:158-160.
6. van Wiechen MP, Tchétché D, Ooms JF, et al. Suture- or Plug-Based Large-Bore Arteriotomy Closure:A Pilot Randomized Controlled Trial. JACC Cardiovasc Interv. 2021;14:149-157.
7. Sohal S, Mathai SV, Nagraj S, et al. Comparison of Suture-Based and Collagen-Based Vascular Closure Devices for Large Bore Arteriotomies —A Meta-Analysis of Bleeding and Vascular Outcomes. J Cardiovasc Dev Dis. 2022;9:331-342.
8. Sedhom R, Dang AT, Elwagdy A, et al. Outcomes with plug-based versus suture-based vascular closure device after transfemoral transcatheter aortic valve replacement:A systematic review and meta-analysis. Catheter Cardiovasc Interv. 2023;101:817-827.
9. Van Mieghem NM, Latib A, van der Heyden J, et al. Percutaneous plug-based arteriotomy closure device for large-bore access:a multicenter prospective study. JACC Cardiovasc Interv. 2017;10:613-619.
10. Wood DA, Krajcer Z, Sathananthan J, et al. SAFE MANTA Study Investigators. Pivotal clinical study to evaluate the safety and effectiveness of the MANTA percutaneous vascular closure device. Circ Cardiovasc Interv. 2019;12:e007258.
11. Dumpies O, Kitamura M, Majunke N, et al. Manta versus Perclose ProGlide vascular closure device after transcatheter aortic valve implantation:initial experience from a large European center. Cardiovasc Revasc Med. 2022;37:34-40.
12. Abdel-Wahab M, Hartung P, Dumpies O, et al. Comparison of a Pure Plug-Based Versus a Primary Suture-Based Vascular Closure Device Strategy for Transfemoral Transcatheter Aortic Valve Replacement:The CHOICE-CLOSURE Randomized Clinical Trial. Circulation. 2022;145:170-183.
13. Martinho S, Jorge E, Marinho V, Baptista R, Costa M, Gonçalves L. The MANTA vascular closure device in transfemoral TAVI:a real-world cohort. REC Interv Cardiol. 2024;6:7-12.
Are the radiation doses we use in interventional procedures appropriate? Cardiologists should be able to answer this question, which is particularly important in pediatric patients. However, the answer matters not only to patients but also to the health professionals involved in these procedures. The occupational radiation doses received by health staff are associated with the doses received by patients, and “optimization” (keeping radiation doses to the minimum needed to achieve the clinical objective of the procedures involved) should be managed comprehensively for patients and professionals alike.1
The International Commission on Radiological Protection (ICRP) recommends using “diagnostic reference levels” (DRLs) to help in the optimization of imaging modalities with ionizing radiation (including interventional procedures).2
DRLs are indicative of “good clinical practice”. It is recommended that they be established for specific clinical indications and can be estimated for the local, national, or regional level by using the third quartile of the distribution of the median values of the dose indicators for patients from various centers representative of these clinical practices.2
The term “achievable dose value” has been proposed in the United States for the 50th percentile instead of the third quartile. Although the ICRP has stated that the median could be used as an additional step in optimization, the recommendation of using the third quartile recommendation to estimate DRLs still stands.2
For interventional procedures, the most widely used radiological measure is the kerma-area product (KAP), which is numerically equivalent to the dose-area product (DAP), and serves as one of the main indicators of the radiation doses received by patients. Secondary indicators that can also be used are the kerma at the patient entrance reference point (15 cm below the isocenter), fluoroscopy time, and the number of cine images acquired. These latter 2 indicators are becoming less relevant because doses depend on different image acquisition modes.
The ICRP recommends taking into consideration the complexity of interventional procedures, since it can increase DRLs significantly. Because complexity can vary widely for a single procedure, carried out for the same or similar clinical indications, it is important to assess its impact on the doses delivered to patients.3,4
The ICRP recommendations have been included in the European regulations (Directive 59/2013 EURATOM)5 and the corresponding practical guidelines of the European Union.6,7 For pediatric procedures, it is suggested that DRLs be estimated based on patient age and weight categories.2
The radiation doses received by pediatric patients vary widely depending on their size and weight. Although variations are inevitable, we should try to avoid those stemming from inappropriate use of imaging modalities (different fluoroscopy or cine modes) or protocols. DRLs help optimize radiation protection.
Different fluoroscopy and cine modes with varying dose rates (and image quality) can be used, substantially impacting the radiation doses received by patients. Factors that play a key role in delivered radiation doses are collimation, C-arm x-ray machine angles, fluoroscopy sequence recording to save cine sequences, and rotational acquisitions.
Ways to significantly reduce the radiation doses received by patients and health professionals are knowing the quality control results of x-ray machines (to understand dose differences between cine and fluoroscopy acquisitions) and fostering collaboration between hospital radiologists and cardiologists, along with continuous medical education programs on radiation safety.
All these variables associated with different operating modes can substantially change the doses delivered to patients and the quality of diagnostic information. Therefore, cardiologists’ knowledge and experience of their imaging equipment are crucial. In general, state-of-the-art machines reduce radiation doses while maintaining similar or improved diagnostic information. Quantifying all these factors and deciding whether corrective actions are needed involves comparing radiation doses for specific procedures with the DRLs.
The Royal Decree that transposes part of the European Directive to the Spanish legislation8 demonstrates the implementation and regular review of DRLs. If these DRLs are consistently and significantly exceeded, or if image quality deteriorates repeatedly, the corresponding local reviews should be undertaken and appropriate corrective measures should be implemented without delay.
Some automated dose management systems allow real-time reception and processing of the radiation doses received by patients and operators. These systems can create alerts for safer interventional practices.9,10
Several studies have been published on DRLs in interventional cardiology for adult patients (DOCCACI program) in Spain11,12 with collaboration from the Spanish Society of Cardiology.
No nationwide results have been published on the doses received by pediatric patients in interventional cardiology until now. However, Rueda Núñez et al.13 recently presented the results of the Radcong-21 Registry conducted by the Cardiac Catheterization Working Group of the Spanish Society of Pediatric Cardiology and Congenital Heart Disease (GTH-SECPCC) on the overall values from a sample of 1090 procedures across 10 different hospitals. This registry represents a significant initiative that could encourage other centers to compare their values with dose indicators obtained from a representative sample of multiple Spanish hospitals in patients with congenital heart disease treated with cardiac catheterization and categorized by type of procedure and estimated radiation risk (ERR).
The study authors used medians, although DRL values refer to the third quartile of the distribution of the median values in the different centers involved. Specific DAP/kg values are provided for certain specific procedures to treat prevalent conditions such as aortic coarctations, atrial septal defects, ductus arteriosus occlusions, aortic and pulmonary valvuloplasties and pulmonary valve implantations following the methodology proposed by Quinn et al.14 in the United States.
DAP/kg/fluoroscopy is a parameter that can be confusing when comparing radiation doses. This is because the total DAP includes contributions from fluoroscopic imaging—corresponding to different fluoroscopy modes with very different dose values—and cine acquisitions.
To facilitate comparisons and potential optimization efforts, the authors could provide dose indicator values (DAP/kg) tailored to a wider range of procedure types in future updates of the results. They could also use the third quartiles of the distribution of the median values for each center for the weight categories recommended by the ICRP and European guidelines.2,6,7
We could speculate whether it would be better to perform a global analysis across groups of different procedures or an analysis specifically designed for procedures with specific clinical indications. Quinn et al.14 choose the former, while managing the DAP/kg values as the primary dose parameter. However, a global approach does not allow analysis of specific procedures requiring corrective measures when the doses delivered to some patients may be very high. These doses exceeding the “good clinical practice” threshold can be used in certain procedures, but not in others, within the 3 REC (Radiation Exposure Category) groups proposed by Quinn et al.14 in their methodology. The advantage of using DRLs per weight group is that these DLRs are estimated for specific clinical indications, thus enabling easy comparisons with the dose indicators used in different hospitals for these procedures.
The global KAP/kg values for groups of different procedures may be balanced if there are procedures using higher radiation doses than necessary (due to excessive cine acquisitions, high-dose fluoroscopy modes, lack of collimation, etc) and other procedures requiring standard doses. This could indicate overall improvement (fewer doses in procedural groups), but does not necessarily indicate improvement in all types of procedures.
The effort made by the GTH-SECPCC to obtain and process PDA/kg values represents a significant advancement that could be further expanded in the future. This could involve establishing initial DRLs (KAP values) in Spain, based on weight and age groups, following the recommendations of the European guidelines and ICRP.
FUNDING
None.
CONFLICTS OF INTEREST
None reported.
REFERENCES
1. ICRP. Occupational radiological protection in interventional procedures. ICRP Publication 139. Ann. ICRP 2018 47. Available at: https://www.icrp.org/publication.asp?id=ICRP%20Publication%20139. Accessed 17 Jun 2023.
2. ICRP. Diagnostic reference levels in medical imaging. ICRP Publication 135. Ann. ICRP 2017. Available at: https://www.icrp.org/publication.asp?id=ICRP%20Publication%20135. Accessed 17 Jun 2023.
3. Bernardi G, Padovani R, Morocutti G, et al. Clinical and technical determinants of the complexity of percutaneous transluminal coronary angioplasty procedures:analysis in relation to radiation exposure parameters. Catheter Cardiovasc Interv. 2000;51:1-9;discussion 10.
4. Balter S, Miller DL, Vano E, et al. A pilot study exploring the possibility of establishing guidance levels in x-ray directed interventional procedures. Med Phys. 2008;35:673-680.
5. EUR-LEX. Council directive 2013/59/Euratom of 5 December 2013 laying down basic safety standards for protection against the dangers arising from exposure to ionising radiation and repealing Directives 89/618/Euratom, 90/641/Euratom, 96/29/Euratom, 97/43/Euratom and 2003/122/Euratom. Official Journal of the European Union. Available at: https://eur-lex.europa.eu/eli/dir/2013/59/oj. Accessed 3 Jul 2023.
6. Radiation Protection No 185 - European Guidelines on Diagnostic Reference Levels for Paediatric Imaging. Directorate-General for Energy Directorate D. Radiation Protection and Nuclear Safety. 2018. Available at: https://energy.ec.europa.eu/system/files/2018-09/rp_185_0.pdf. Accessed 17 Jun 2023.
7. Radiation Protection 195. European study on clinical diagnostic reference levels for X-ray medical imaging EUCLID. Luxembourg:Publications Office of the European Union, 2021. Available at: European study on clinical diagnostic reference levels for X-ray medical imaging - Publications Office of the EU (europa.eu). Accessed 3 Jul 2023.
8. Real Decreto 601/2019, de 18 de octubre, sobre justificación y optimización del uso de las radiaciones ionizantes para la protección radiológica de las personas con ocasión de exposiciones médicas. Boletín Oficial del Estado núm. 262, de 31 de octubre de 2019. Available at: https://www.boe.es/eli/es/rd/2019/10/18/601. Accessed 19 Jun 2023.
9. Vano E, Sanchez RM, Fernandez JM. Strategies to optimise occupational radiation protection in interventional cardiology using simultaneous registration of patient and staff doses. J Radiol Prot. 2018;38:1077-1088.
10. Vano E, Fernandez-Soto JM, Ten JI, Sanchez Casanueva RM. Occupational and patient doses for interventional radiology integrated into a dose management system. Br J Radiol. 2023;96:20220607.
11. Sánchez RM, Vano E, Fernández JM, Escaned J, Goicolea J, PifarréX;DOCCACI Group. Initial results from a national follow-up program to monitor radiation doses for patients in interventional cardiology. Rev Esp Cardiol. 2014;67:63-65.
12. Sánchez R, VañóE, Fernández Soto JM, et al. Updating national diagnostic reference levels for interventional cardiology and methodological aspects. Phys Med. 2020;70:169-175.
13. Rueda Núñez F, Abelleira Pardeiro C, Insa Albert B, et al. Dosimetric parameters in congenital cardiac catheterizations in Spain:the GTH-SECPCC Radcong-21 multicenter registry. REC Interv Cardiol. 2023. https://doi.org/10.24875/RECICE.M23000372.
14. Quinn BP, Cevallos P, Armstrong A, et al. Longitudinal Improvements in Radiation Exposure in Cardiac Catheterization for Congenital Heart Disease:A Prospective Multicenter C3PO-QI Study. Circ Cardiovasc Interv. 2020;13:e008172.
* Corresponding author.
E-mail address: eliseov@med.ucm.es (E. Vañó Carruana).
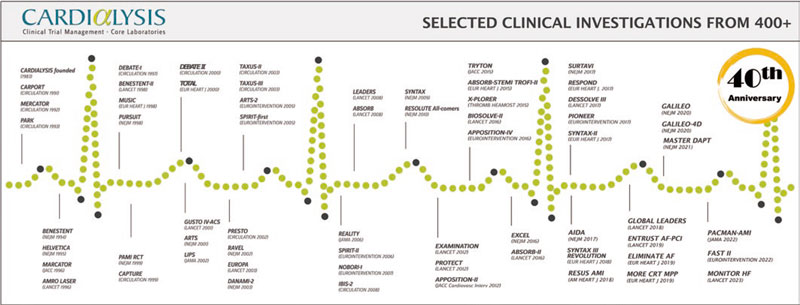
Cardialysis was founded in 1983 by visionary professionals from the Thoraxcenter at Erasmus University Medical Center (EMC) in Rotterdam, The Netherlands. This initiative emerged to address the need for a specialized research organization able to plan, execute, and report European cooperative clinical investigations in the field of cardiovascular research.1 The mission of Cardialysis is “to be at the heart of cardiovascular research” in the fullest sense of the phrase. To achieve this mission, the organization strives to collaborate with clinicians, trialists, research professionals, regulators, industry partners, and research organizations that share the same passion. Throughout the first 40 years, its well-established reputation has been based on dedication, diligence, and industry-leading standards. This has been made possible by attracting and retaining talented employees, as well as by cultivating long-standing relationships with investigators, clients, and partners.
Organizations similar to Cardialysis are typically classified as either academic research organizations (AROs) or contract research organizations (CROs). AROs, such as clinical trial units and epidemiology departments, are typically affiliated with universities or university hospitals, and usually design and manage investigator-initiated single-center or multicenter national clinical trials. These organizations are academically-driven and their traditional objectives are to facilitate research and education, publish innovative research in peer-reviewed journals, and support PhD programs. In contrast, CROs are private entities performing sponsor-driven clinical trials across all phases of clinical development. These organizations are business-driven, and expected to remain self-sustaining by establishing research contracts with the medical industry in a strict regulatory environment.
Similar to other world-leaders in cardiovascular research, Cardialysis has successfully adopted a hybrid ARO/CRO model, combining the best of the 2 models. First, the organization participates in innovative research that has played a pivotal role in the development and improvement of therapies in cardiology. This involvement has resulted in numerous high-impact publications and the completion of more than 100 theses through its support of in-house academic research. Figure 1 lists notable trials in which Cardialysis participated, including research in coronary artery disease, structural heart disease, heart failure, hypertension, and peripheral artery disease. Second, the organization proudly maintains a global network of renowned investigators who play key roles in the academic leadership of trials (eg, within steering committees), independent clinical events committees (CECs),2 independent data and safety monitoring boards,3 and imaging core lab supervision. Third, Cardialysis operates as a private, independent entity that serves both university hospitals worldwide, as well as leading pharmaceutical and medical device industry partners. Fourth, the organization maintains state-of-the-art infrastructure and follows standardized procedures, ensuring efficiency and quality. Finally, Cardialysis consistently adheres to applicable regulatory requirements for trial execution in all active regions, including Europe, the United States of America, and China.
Figure 1. Timeline of landmark trials conducted with Cardialysis.
THE CARDIALYSIS CORE LAB
Over the past half century, clinical research has had an enormous impact on clinical outcomes, which was eloquently summarized by Nabel and Braunwald.4 These advancements are inextricably linked to the development of therapeutic and diagnostic devices, especially in interventional cardiology. The development of coronary angioplasty involved progressive iterations in coronary stent technologies and continuous innovation in intracoronary imaging.5 Similarly, advancements in transcatheter therapies for aortic, mitral, and tricuspid conditions have paralleled the progress in imaging modalities and techniques.6 Independent and consistent assessments of imaging outcomes are an important component of dossier evaluations when commercialization approvals are sought for new devices.7
Cardialysis started as a central reading facility for electrocardiography-Holter monitoring. However, over time it has validated and implemented rigorous analysis methodologies for various imaging techniques within the coronary core lab. These include quantitative coronary angiography (n = 67 000+), intravascular ultrasound (n = 16 000+), near-infrared spectroscopy (n = 1500+), and optical coherence tomography (n = 5000+). Notably, Cardialysis also launched and maintains the globally used SYNTAX score (n = 10 000+) website.8 Due to a shift toward structural heart research, its echocardiography core lab has become its largest laboratory and serves large European and global investigations (n = 39 000+). Additionally, the organization operates magnetic resonance imaging (n = 1000+), cardiac computed tomography (n = 1000+), and electrocardiography-Holter core laboratories (n = 270 000+).
CARDIALYSIS CLINICAL TRIAL ACTIVITIES
The workload involved in a clinical investigation should not be underestimated. For example, the ongoing IVUS CHIP randomized clinical trial (NCT04854070) involves 40 sites in Europe and more than 200 professionals in the execution of the study, excluding the members of the Ethics Committees. Managing and coordinating more than 200 professionals across 7 countries for a minimum of 5 years requires high levels of availability, dedication, consistency, and well-established standardized procedures. Having an all-inclusive research organization performing ambitious trials increases efficiencies. Table 1 lists the activities performed at Cardialysis, which are those expected of any all-round professional research organization. Further information is available on the Cardialysis website.8
Table 1. List of activities performed at a cardiovascular research organization
Trial design and protocol development |
Steering committee set-up and coordination |
Site feasibility and selection |
Site start-up and regulatory submissions |
Site contracting |
Project management |
Monitoring management |
Site management and site monitoring |
Safety reporting |
Medical monitoring |
Electronic case report forms development and hosting |
Data management |
Clinical events committee set-up and coordination |
Data and safety monitoring board set-up and coordination |
Publication committee set-up and coordination |
Biostatistics: sample sizing, statistical analysis plan, and statistical reporting |
Medical writing including patient narratives |
Publication strategy |
Quality assurance and regulatory compliance |
THE ACADEMIC RESEARCH CONSORTIUM
In 2006, with the increasing need for consistent endpoint definitions in coronary artery disease research, and specifically due to the challenges posed by the classification of stent thrombosis, leading AROs in the United States and Europe, including Cardialysis, collaborated to found the Academic Research Consortium (ARC).8 The primary mission of the ARC is to promote informed and collaborative dialogue among stakeholders, with the goal of developing consensus definitions and nomenclature for targeted areas of new medical device development, and to disseminate such definitions in the public domain.8 The first initiative focused on endpoint definitions and classifications for coronary intervention trials and was published in 2007.9 This consensus document was developed in consultation with regulatory agencies in both the United States and Europe, and became one of the most cited articles in interventional cardiology. To date, the ARC has successfully launched and completed 20 programs and is currently running 15 new initiatives based on an unprecedented level of global scientific collaboration.8
EUROPEAN CARDIOVASCULAR RESEARCH INSTITUTE
Europe is known for its stringent regulations on academic research. Importantly, the sponsor of a clinical investigation is the legal entity (or individual) that ensures that regulations are met and that monitors patient safety either directly or through a data and safety monitoring board. The sponsor holds full rights to the study data (ie, has sole ownership) and is responsible for verifying data integrity, ensuring that published results are consistent with the locked final analysis database. Although prominent academic centers possess the expertise to sponsor clinical trials, the size of certain studies requires external support for manageability.
In response to this need, the European Cardiovascular Research Institute (ECRI) was founded in 2012 by the Cardialysis group as an academic research platform capable of overseeing the execution of large, multicenter clinical trials and of fulfilling sponsor responsibilities. Since April 2023, the ECRI has become an independent foundation (Stichting) under Dutch law. This institute combines 3 elements of success: its outstanding academic leadership represented by its pro bono scientific advisory board (figure 2), a well-established network of investigators and research professionals, and the possibility to conduct clinical research activities in partnership with Cardialysis. Through this collaborative model, the ECRI-Cardialysis joint venture has successfully conducted some of the largest interventional cardiology trials, with the GLOBAL LEADERS trial being the most representative.10 This trial enrolled 15 968 patients at 130 sites across 18 countries. Another example is the MASTER DAPT trial,11 which enrolled 4579 high-bleeding risk patients at 140 sites in 30 countries.
Figure 2. European Cardiovascular Research Institute foundation and its scientific advisory board. ECRI, European Cardiovascular Research Institute.
The ECRI-Cardialysis partnership is currently conducting 3 landmark investigations on the use of coronary imaging and coronary physiology to guide percutaneous coronary interventions. Up-to-date details are available on the Cardialysis website.8
A LANDMARK FIGURE
Numerous clinicians and clinical research professionals have passed through the door of Cardialysis, with some staying for a lifetime and others for a short period. Undoubtedly, the most salient contributor to the organization’s innovations and successes has been Prof. Patrick W. Serruys. His mentor, Prof. Paul Hugenholtz, acknowledged as the father of the European Society of Cardiology, and one of the founding members of Cardialysis, recognized Prof. Serruys as a natural talent and luminary in clinical research. Prof. Serruys joined Cardialysis during its early days and since then played a decisive role in the organization. His innovations and contributions included the development of coronary imaging techniques (eg, he was coinventor of quantitative coronary angiography); the establishment of best practices in interventional clinical trials, including the design and execution of the BENESTENT study,12 which was the first of its kind and remains one of the most cited publications in interventional cardiology; research in bioresorbable scaffolds; and an illustrious career that garnered multiple distinctions and awards.13 Although Prof. Serruys left the organization in 2019, his scientific legacy remains highly esteemed.
Currently, a new generation is shaping the present and future of the organization with unwavering commitment to innovation, collaborative research, and, above all, commitment to improved patient outcomes. The 40-year timeline of Cardialysis (figure 1) starts with the CARPORT trial led by Prof. Serruys from the Thoraxcenter, and concludes with the FAST II study led by Dr Joost Daemen14 and the MONITOR HF trial led by Dr Jasper Brugts,15 both also from the Thoraxcenter. While Cardialysis envisions expanding its European and global collaborations in the coming decades, its scientific partnership with EMC has remained strong and will continue to flourish.
THE FUTURE
Cardialysis pledges to continue contributing to the design, execution and reporting of clinical trials as an independent and scientifically-driven cardiovascular research organization and core laboratory. Its priorities are patient safety, data integrity, and the production of high-quality data, which have been achieved by committed employees, passionate investigators, and long-standing collaboration with its valued partners.
Additionally, Cardialysis remains committed to further advancing continued standardization of clinical trial definitions, design principles, and core laboratory methods, given that standardization has proven to be a catalyst in clinical research. Current innovations at Cardialysis focus on implementing artificial intelligence, streamlining clinical trial processes, harnessing the potential of real-world data, and mastering applicable regulatory science.
FUNDING
None.
CONFLICTS OF INTEREST
E. Spitzer is a board member and shareholder of Cardialysis and a board member of the ECRI.
ACKNOWLEDGMENTS
Our 40th anniversary is dedicated to our beloved colleague Eline Montauban van Swijndregt†.
REFERENCES
1. Organization, review, and administration of cooperative studies (Greenberg Report):a report from the Heart Special Project Committee to the National Advisory Heart Council, May 1967. Control Clin Trials. 1988;9:137-148.
2. Spitzer E, Fanaroff AC, Gibson CM, et al. Independence of clinical events committees:A consensus statement from clinical research organizations. Am Heart J. 2022;248:120-129.
3. United States Food and Drug Administration. Guidance for Clinical Trial Sponsors. Establishment and Operation of Clinical Trial Data Monitoring Committees. March 2006. Available at: https://www.fda.gov/regulatory-information/search-fda-guidance-documents/establishment-and-operation-clinical-trial-data-monitoring-committees. Accessed 30 June 2023.
4. Nabel EG, Braunwald E. A tale of coronary artery disease and myocardial infarction. N Engl J Med. 2012;366:54-63.
5. van Zandvoort LJC, Ali Z, Kern M, van Mieghem NM, Mintz GS, Daemen J. Improving PCI Outcomes Using Postprocedural Physiology and Intravascular Imaging. JACC Cardiovasc Interv. 2021;14:2415-2430.
6. Casenghi M, Popolo Rubbio A, Menicanti L, Bedogni F, Testa L. Durability of Surgical and Transcatheter Aortic Bioprostheses:A Review of the Literature. Cardiovasc Revasc Med. 2022;42:161-170.
7. United States Food and Drug Administration. Clinical Trial Imaging Endpoint Process Standards. Guidance for Industry. April 2018. Available at: https://www.fda.gov/regulatory-information/search-fda-guidance-documents/clinical-trial-imaging-endpoint-process-standards-guidance-industry. Accessed 30 June 2023.
8. Cardialysis. Available at: www.cardialysis.nl/library/links. Accessed 2 July 2023.
9. Cutlip DE, Windecker S, Mehran R, et al;and Academic Research C. Clinical end points in coronary stent trials:a case for standardized definitions. Circulation. 2007;115:2344-2351.
10. Vranckx P, Valgimigli M, Juni P, et al;and Investigators GL. Ticagrelor plus aspirin for 1 month, followed by ticagrelor monotherapy for 23 months vs aspirin plus clopidogrel or ticagrelor for 12 months, followed by aspirin monotherapy for 12 months after implantation of a drug-eluting stent:a multicentre, open-label, randomised superiority trial. Lancet. 2018;392:940-949.
11. Valgimigli M, Frigoli E, Heg D, et al;and Investigators MD. Dual Antiplatelet Therapy after PCI in Patients at High Bleeding Risk. N Engl J Med. 2021;385:1643-1655.
12. Serruys PW, de Jaegere P, Kiemeneij F, et al. A comparison of balloon-expandable-stent implantation with balloon angioplasty in patients with coronary artery disease. Benestent Study Group. N Engl J Med. 1994;331:489-495.
13. Serruys DP. Featuring:Dr Patrick Serruys. Eur Cardiol. 2018;13:80-82.
14. Masdjedi K, Tanaka N, Van Belle E, et al. Vessel fractional flow reserve (vFFR) for the assessment of stenosis severity:the FAST II study. EuroIntervention. 2022;17:1498-1505.
15. Brugts JJ, Radhoe SP, Clephas PRD, et al;investigators M-H. Remote haemodynamic monitoring of pulmonary artery pressures in patients with chronic heart failure (MONITOR-HF):a randomised clinical trial. Lancet. 2023;401:2113-2123.
Subcategories
Editorials
Percutaneous coronary intervention of the left main in the elderly: a reasonable option
Department of Cardiology and Angiology, University Heart Center Freiburg · Bad Krozingen, Medical Center – University of Freiburg, Faculty of Medicine, University of Freiburg, Freiburg, Germany
Original articles
Editorials
Are we ripe for preventive percutaneous coronary interventions?
aDepartment of Cardiology, McGill University Health Center, Montreal, Quebec, Canada
bDepartment of Structural Heart Disease, Silesian Medical University, Katowice, Poland
Original articles
Debate
Debate: Preventive coronary intervention for vulnerable plaque
The clinical cardiologist’s approach
Servicio de Cardiología, Hospital Universitario de Jaén, Jaén, Spain
The interventional cardiologist’s approach
Departamento de Cardiología, Hospital Universitari de Bellvitge, Institut d’Investigació Biomèdica de Bellvitge (IDIBELL), Universitat de Barcelona, L’Hospitalet de Llobregat, Barcelona, Spain