Available online: 09/04/2019
Editorial
REC Interv Cardiol. 2020;2:310-312
The future of interventional cardiology
El futuro de la cardiología intervencionista
Emory University School of Medicine, Atlanta, Georgia, United States
Regulation (EU) 2017/745 of the European Parliament and of the Council of 5 April 2017 was published to regulate medical devices with the aim of bolstering the safety, quality, and efficacy of medical products in Europe.1
The regulation covers medical products intended for the diagnosis and treatment of numerous cardiovascular conditions, including high-risk devices such as pacemakers, defibrillators, artificial hearts, stents, cardiovascular sutures, heart valves, catheters, cardiovascular wires, and cardiac ablation instruments. According to the classification rules outlined in the regulation, all these high-risk medical products are classified as Class III.
The process for obtaining the CE mark for a medical device requires the manufacturer to demonstrate that the product meets the established safety and performance requirements and to conduct a clinical evaluation to validate the intended indication and purpose of use. For Class III product certification, a notified body designated by a member state authority must verify that the manufacturer has the objective technical and clinical documentation required to demonstrate that the product meets all the claims made by the manufacturer on the product. The authorized body issues a “CE Declaration of Conformity” including the manufacturer’s information, the product’s unique identifier, class, intended purpose, test reports and documentation, date and issue of validity, and details of the notified body involved in the process of granting the CE mark. The manufacturer’s company must also implement a quality management system to ensure that the manufactured products meet specified standards. After auditing the manufacturer’s facilities, the body issues an “EU quality management system certificate” detailing the scope of the quality system and type of manufactured products.
In other words, for the marketing of Class III medical products, the manufacturer must hold 2 different EU certificates issued by a notified body: one for the product and one for the quality management system.
Health care workers or users of a medical product can easily identify which notified body participated in its assessment by checking the product label, which is identified by a 4-digit number appearing alongside the CE mark. The name of the organization behind that number can be found on the European Commission’s website.2 For example, if the digits 0318 appear next to the CE mark on the label or the instructions for use of a medical product, it indicates that the evaluation was conducted by the National Certification Center for Medical Product, the sole notified body designated by the Ministry of Health.
The main change introduced in the regulation on product requirements involves the clinical evaluation. The assessment is especially strict for Class III products, which, as previously mentioned, are high risk. The first requirement is that the clinical evaluation validating the indication for use must be based on clinical data obtained from clinical investigations conducted with the product itself or a product that is technically, biologically, and clinically equivalent. The second requirement is that manufacturers must have access to the primary clinical data supporting the clinical evaluation of the medical product in question, either because they own them, or because the data have been published, or because they have a contractual agreement with the owner allowing permanent access and availability.
Although it may seem trivial, since the publication of the regulation, the availability of a compliant clinical evaluation has been the Achilles’ heel for manufacturers of medical products intending to market their products in Europe in the coming years.
During the 3 decades since the implementation of the directives, special emphasis has been placed on ensuring the safety and quality of medical products, while the available objective evidence supporting their clinical benefit has been relegated to a secondary role. Consequently, manufacturers of medical products that have been on the market for years have had to make considerable efforts and investments to obtain sufficient clinical data with the necessary level of evidence to support the clinical risk-benefit ratio esta- blished by the new legislation. Many have had to devise new clinical evaluation plans or review existing ones, including conducting specific postmarket clinical follow-up studies to provide clinical data with an adequate level of evidence. Therefore, we could say that a culture of the need for clinical research and publication of the obtained data is emerging in the medical products sector.
On the other hand, to minimize potential discrepancies between notified bodies in the assessment of the clinical evaluation of Class III implantable medical products (such as pacemakers), the regulation has established a centralized supervision procedure by a panel of experts in medical products from the European Medicines Agency (EMA). The role of this panel is to review and confirm the adequacy of both the clinical evaluation conducted by the manufacturer and the assessment made by the notified body, and provide any recommendations deemed appropriate regarding the decision on certifying the medical product. These recommendations may include proposing to certify or not certify the product, or to limit or restrict indications, among others.
An interesting point is that 18 out of the 43 applications received by the panel so far correspond to medical products within the “circulatory system” clinical area. In particular, the clinical evaluations of some stents, implantable defibrillators, and various types of heart valves have already undergone this procedure, and the resulting public opinions can be consulted in a list within the framework of the European Commission’s clinical evaluation consultation procedure.3
In addition, manufacturers of these types of products can seek guidance from the panel of experts before starting clinical development to confirm that the strategy designed for clinical development is appropriate and ensure that the resulting clinical evaluation will fully comply with the current legislation. If manufacturers decide to submit this voluntary query, the response issued by the panel will be binding. In other words, manufacturers will not be able to implement a different clinical evaluation plan from that recommended by the panel if they want to obtain the CE mark for the product.
The cornerstone of the CE certification model described is the competence of the personnel conducting the evaluation tasks. The personnel involved in the process of conducting or assessing the clinical evaluation of a medical product must have adequate knowledge. At the forefront of this chain are the manufacturers because they have had to review the competence of their staff to ensure that clinical evaluations are conducted by personnel experienced in clinical evaluation, competent in bibliographic searches, and with sufficient clinical knowledge and use of the products. Next are the notified bodies, which have to ensure that they have sufficient personnel with relevant clinical knowledge to issue a clinical judgment on the product’s risk-benefit ratio after analyzing and scientifically testing the clinical data collected in the clinical evaluation provided by the manufacturers. Furthermore, clinicians internal to the notified bodies must verify that the personnel conducting the clinical evaluations provided by the manufacturers are qualified to perform the task. Further along the review chain, notified bodies are audited by European teams of qualified professionals, who, in turn, must verify that the competencies of the personnel conducting the assessments of the clinical evaluations in the notified bodies meet the criteria of experience and training established in the regulation.
This regulation also encourages the manufacturers of medical products to hire health care workers with clinical experience, who have their own opinions on the products they use in their routine clinical practice. These professionals can participate in the early stages of product design, engage in usability testing, and, naturally, as occurs with drugs, promote clinical research both before and after product marketing. This helps to confirm the clinical benefit of medical products throughout their life cycle.
Health care workers must be aware of the value of their experience and clinical knowledge in ensuring that the medical products entering the market are truly innovative and meet the needs of patients.
The responsible and committed contribution made by each of the parties involved in conducting and reviewing the clinical evaluation of medical products will, on the one hand, provide greater assurance of the rigor, robustness, and sufficiency of the clinical data supporting a product’s indication. On the other hand, it will serve to standardize the criteria applied in the evaluation and ensure that the level of evidence required for all medical products bearing the CE mark under the new regulation is the same. These measures will restore confidence in the legislative model of medical products, ensuring that all manufacturers marketing their medical products in the European common market play by the same rules. Therefore, that the CE certification under which products are marketed will provide identical safeguards to patients, regardless of the country of origin, manufacturer, or issuing body.
FUNDING
None declared.
STATEMENT ON THE USE OF ARTIFICIAL INTELLIGENCE
No artificial intelligence has been used in the preparation of this article.
CONFLICTS OF INTEREST
None declared.
REFERENCES
1. Parlamento Europeo. Reglamento (UE) 2017/745 del Parlamento Europeo y del Consejo, de 5 de abril de 2017, sobre los productos sanitarios, por el que se modifican la Directiva 2001/83/CE, el Reglamento (CE) n.º178/2002 y el Reglamento (CE) n.º1223/2009, y por el que se derogan las Directivas 90/385/CEE y 93/42/CEE del Consejo. DOUE. 2017;117:1-175.
2. European Commission. New Approach Notified and Designated Organisations -NANDO. Available at: https://webgate.ec.europa.eu/single-market-compliance-space/%23/notified-bodies/notified-body-list?filter=legislationId%3A34%2CnotificationStatusId%3A1. Accessed 18 Dec 2023.
3. European Commission. List of opinions provided under the CECP. Available at: https://health.ec.europa.eu/medical-devices-expert-panels/experts/list-opinions-provided-under-cecp_en#p2.
* Corresponding author.
E-mail address: ghernandez@certificaps.gob.es (G. Hernández Hernández).
INTRODUCTION
When scientific projects or articles are evaluated, objections are often raised that may prevent their performance or publication. Sometimes, the flaws noted may not be correct or relevant to the study. In this article, we review the most common types of objections that can hinder the progress of medical research and suggest ways to reduce them.
CLINICAL (OR PROCEDURAL) OBJECTIONS AND STATISTICAL/METHODOLOGICAL OBJECTIONS
The objections an evaluator can make to a research project can be grouped into 2 broad categories: clinical (or procedural) and statistical/methodological.
The former can be addressed and, if necessary, refuted by the author of the project as they relate to the clinical problem per se. In this regard, the author of the project has more expertise and sometimes more up-to-date knowledge than the evaluator on the issue in question. A common example could be the objection, “the project does not specify under which conditions baseline blood pressure should be measured, or the criteria chosen to define hypertension.” The researcher can acknowledge the flaw in his/her protocol and correct it or argue that the objection is incorrect.
The situation is different with statistical/methodological objections. Researchers, whether acting as evaluators or persons who are evaluated, are not usually experts in research methodology and biostatistics. Below are a few examples of this type of objection.
Common erroneous statistical/methodological objections
Sample size
Contrary to what most researchers believe, the objection of an insufficient sample size is only relevant in highly specific situations. In some cases, it is not accurate; for example, if the result has a very small P value that constitutes strong evidence against the null hypothesis. It does not make any sense either in somewhat more complex situations.1
Statistical power
Statistical power depends on 4 parameters, whose value is often not predefined, so by choosing suitable values for these parameters, researchers can obtain almost any value for statistical power. In fact, when researchers are asked about the figure for statistical power, it is often insufficient to give a specific value, because the values of other parameters associated with such power are also necessary. Moreover, it is obvious that by slightly modifying these values within reasonable ranges, very different power values can be obtained.2
Test on the normal distribution of the response variable
In many cases, this objection may be doubly mistaken: either because the response variable is dichotomous and will be treated as such in the analysis, or because the sample size used is greater than, say, 30, and the central limit theorem guarantees a very good approximation to the normal distribution of the statistic used in the test. Naturally, it can never be guaranteed whether the variable has a normal distribution or not. Thus, in cases with a confirmed lack of normal distribution, the robustness of some parametric tests vs nonnormality must be taken into account.3 In cases with a strong association and an extremely small P value in the test, it should be noted that if the true P value of the test were, say, 10 times larger or 10 times smaller than that found in the parametric test, the practical conclusion would be the same.
Control group and study validity
While a control group is a great asset in many situations, demanding its presence should not be a universally or undisputed mantra. In some situations—and when used appropriately—historical controls provide enough information to draw very interesting conclusions. In other cases, each patient serves as his/her own control, thus allowing the use of intraindividual variability, which is often less than interindividual variability and, therefore, provides more powerful tests in many cases.
Pilot trials
Randomized clinical trials (RCTs) add highly useful methodological refinements to effectively determine the safety and efficacy profile of a new drug or procedure. However, pilot trials can add these same methodological refinements and be controlled, randomized, and blinded to a point that the level of scientific evidence they provide can be equivalent to that of RCTs, with significant advantages regarding time and cost savings. In addition, in general, their size is not a determining factor that compromises their validity. Then, what is the main difference between the 2 designs? The difference lies not in the level of evidence they provide, but in the administrative process involved. RCTs require approval from external hospital, regional, or national committees, while pilot trials are endorsed by the expertise of the medical team involved in their design. For external evaluators, it is more challenging to make accurate assessments of each aspect of the project and provide a sound judgment. Moreover, if they have the authority to veto the study, there is a possibility of rejecting it based on insufficiently founded considerations.
Observational trials
Blinded RCTs are widely accepted as the best source of evidence on drug and treatment efficacy. However, observational studies can also provide information on long-term safety and efficacy, which is often lacking in RCTs. Additionally, they are less expensive, allow the study of rare events, and provide information more quickly than RCTs. New and ongoing developments in analytical and data technology offer a promising future for observational studies, which already play a key role in researching treatment outcomes. Data from large observational studies can clarify the tolerability profile of drugs and are particularly suitable for large and heterogeneous populations of patients with complex chronic diseases. RCTs and observational trials should, therefore, be considered to complement each other.
Case-control trials
Rothman4 states that case-control trials have gone from “being the Cinderella of medical research to one of its brightest stars.” In case-control designs, it is much more challenging to avoid the distortion caused by confounding factors. However, these issues are partially mitigated by segmentation, matching, and multivariate analysis techniques. In some cases, they can provide significant statistical evidence much faster and more cheaply than clinical trials. Let’s consider an example of a disease that affects 1% of the population who do not follow a particular diet, and 5% of those who do follow it, knowing that 40% of the population follows that diet. A prospective trial would take 80 people from the diet group and another 80 from the control group, and after the agreed-upon time, we would measure the incidence of the disease in each of the 2 groups. The statistical power of this study for an alpha value of 0.05 would be 8%. A case-control trial would take 80 patients with the disease and 80 without it, and with very detailed health records, we would be able to determine the percentage of people who follow that diet in each of the 2 groups. The statistical power would be 93%.
The list of erroneous objections is much longer, however, and each would require a dedicated article to explain it.
CONCLUSIONS
Some of the methodological objections raised by the evaluators are incorrect. In most cases, the evaluated party assumes that his/her project has a major flaw and ends up abandoning it. Consequently, many projects that could have provided valuable information are unfairly discarded slowing down the progress of medicine.
We believe that this anomaly would largely be avoided if: a) evaluators raised methodological objections only in areas in which they have in-depth knowledge; b) whenever possible, the judgment issued by the evaluators from health agencies and bioethics committees was a suggestion instead of a veto; c) the fundamental role of observational trials, which can be highly effective and generally cheaper than clinical trials, was recognized; d) pilot trials were conducted in many cases where they are indicated, because they can be controlled, randomized, and blinded but without the restrictions associated with RCTs (figure 1).
Figure 1. Measures to expedite medical research by promoting the autonomy of qualified physicians, avoiding unjustified methodological objections, and promoting the use of currently underrated designs.
FUNDING
None declared.
CONFLICTS OF INTEREST
None declared.
REFERENCES
1. Egbuchulem KI. The basics of sample size estimation:an editor's view. Ann Ib Postgrad Med. 2023;21:5-10.
2. Prieto-Valiente L, Carazo-Díaz C. Potencia estadística en investigación médica. ¿Quépostura tomar cuando los resultados de la investigación son significativos?Rev Neurol. 2023;77:171-173.
3. Roco-Videla A, Landabur Ayala R, Maureira Carsalade N, Olguín-Barraza M. ¿Cómo determinar efectivamente si una serie de datos sigue una distribución normal cuando el tamaño muestral es pequeño?Nutr Hosp. 2023;40:234-235.
4. Rothman KJ. The origin of Modern Epidemiology, the book. Eur J Epidemiol. 2021;36:763-765.
* Corresponding author.
E-mail address: mmselles@secardiologia.es (M. Martínez-Sellés).
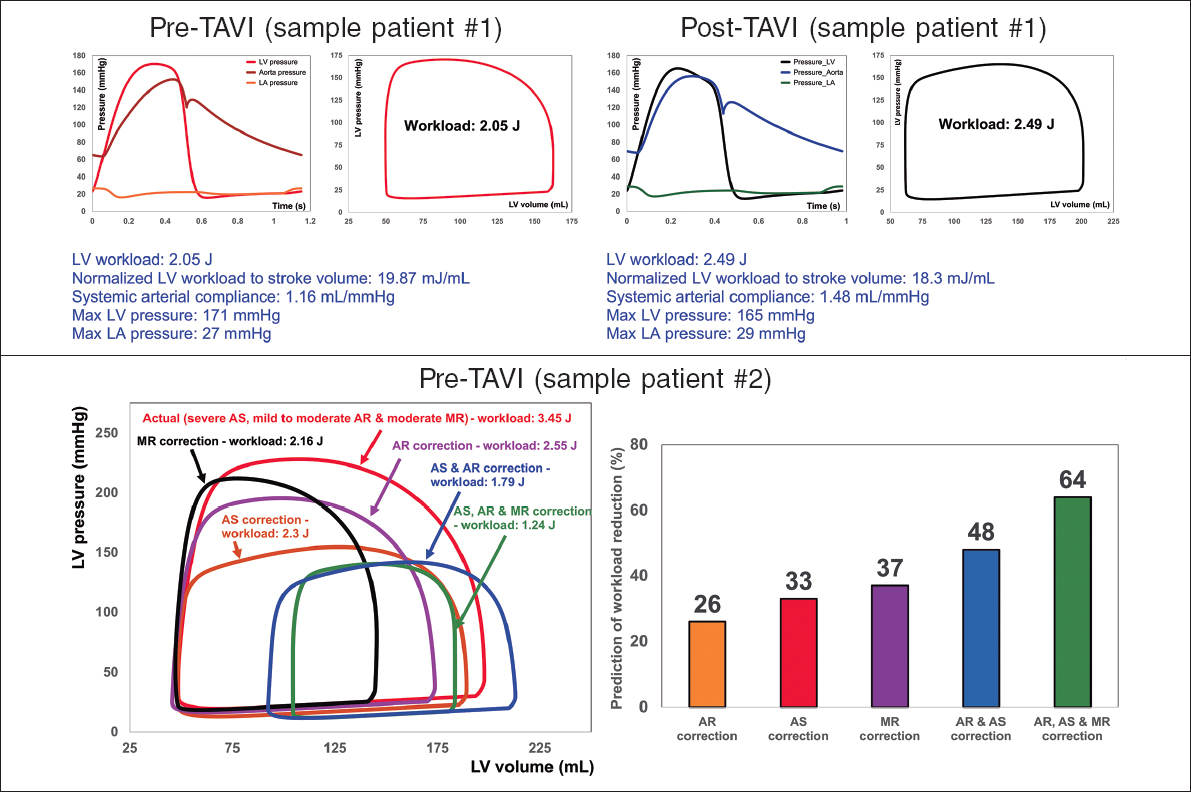
Aortic valve stenosis is one of the most acute and chronic cardiovascular disease conditions. Bicuspid aortic valve is the most common congenital heart abnormality and affected individuals have a 50% chance of developing severe aortic valve stenosis during their lifetime. In aortic valve disease (both aortic valve stenosis and bicuspid aortic valve), the heart valves are damaged and do not work properly. This condition can rapidly affect the pumping action of the heart and can progress to heart failure. Heart failure is a deadly disease affecting at least 26 million people worldwide and its prevalence is increasing with high mortality and morbidity.1 Most importantly, aortic valve disease commonly coexists with other cardiovascular diseases, giving rise to the most general yet fundamentally challenging scenario: complex valvular, ventricular, and vascular diseases (C3VD). In C3VD, multiple valvular, ventricular, and vascular pathologies interact with one another, while the physical phenomena associated with each pathology amplify the effects of others on the cardiovascular system.
Left ventricle (LV) pressure-volume (P-V) loop analysis is a powerful tool to assess cardiac mechanics. This analysis can reveal the pathophysiological mechanisms of heart failure, including heart failure with preserved ejection fraction and myocardial and valvular diseases. It is, therefore, instrumental in the evaluation and management of heart failure and valvular heart disease and can also help explain the short- and long-term effects of valvular and other interventions with cardiac implications. In addition, ventricular P-V loop analysis can be used to monitor the cardiac effects of related medical devices, mechanical heart support, therapeutic interventions, and medications.2-5 Indeed, ventricular P-V loop analysis has exceptional potential for integration into current clinical practice to advance the standard of care.
Aortic valvular disease increases LV pressure, LV end-diastolic pressure, LV workload, the stiffness of the systemic arterial system, and LV afterload, contributing to LV systolic and diastolic dysfunction,6 an important cause of heart failure in such patients. While transcatheter aortic valve implantation (TAVI) provides positive outcomes and has markedly reduced the mortality rate, TAVI fails in nearly 25% to 35% of patients (patients either die or do not recover a reasonable quality of life after the procedure).7 Indeed, the immediate intraprocedural as well the longitudinal hemodynamic changes affecting the aortic-left ventricular system after aortic valve replacement are poorly understood. While TAVI universally reduces the transvalvular pressure gradient, it is anticipated to improve systolic and diastolic LV function in the long-term. Despite the benefits, invasive LV P-V loop analysis revealed impaired LV systolic and diastolic function in the early phase following TAVI.6,8 LV P-V loop analysis also revealed exacerbated heart failure despite successful TAVI procedures in many patients.5,9 Indeed, LV P-V loop analysis elucidated that reductions in transvalvular pressure gradient post-TAVI were not always accompanied by improvements in LV workload. TAVI has been shown to have no effect on LV workload in many patients, while LV workload post-TAVI significantly rose in many others.2,5,10
In clinical settings, cardiac catheterization is the gold standard for evaluating pressure and flow through the heart to perform ventricular P-V loop analysis. However, due to its invasiveness, cost, and high risk, it is impractical for diagnosis in routine daily clinical applications or serial follow-up examinations. Most importantly, cardiac catheterization only provides access to the blood pressure in very limited regions rather than details of physiological flow and pressures throughout the heart and circulatory system. In addition, there is no method to invasively or non-invasively quantify the heart workload that can provide a contribution breakdown of each component of the cardiovascular diseases. This is especially crucial in the presence of TAVI and C3VD, in which quantification of left ventricular workload and its breakdown are important to guide the priority of interventions. Moreover, there is no noninvasive method for determining LV end-diastolic pressure, instantaneous LV pressure, and contractility. All these parameters provide valuable information about the patient’s cardiac deterioration and heart recovery.
Keshavarz-Motamed11,12 developed the first and the only Doppler-based noninvasive patient-specific diagnostic, monitoring, and predictive tool that can investigate and quantify the effects of interventions, medications, and C3VD constituents on the function of the heart and circulatory system at no risk to the patient (figure 1).
Figure 1. Doppler-based patient-specific diagnostic, monitoring, and predictive tool flowchart. The tool uses a few input parameters that can all be measured using Doppler echocardiography simply and reliably. This novel tool4,10–15 was validated against cardiac catheterization and 4D flow MRI in patients with C3VD (so far ~ n = 600) with substantial inter- and intra-patient variability with a wide range of (adult and congenital) cardiovascular diseases. 4D, 4-dimensional; C3VD, complex valvular, ventricular, and vascular diseases; CD, cardiovascular disease; LV, left ventricular; MRI, magnetic resonance imaging.
This novel method4,10-15 offers several key capabilities (figure 2, sample results): a) quantifying details of physiological pulsatile flow and pressures through the heart and circulatory system; b) tracking cardiac and vascular states based on accurate time-varying models that reproduce physiological responses; c) performing LV P-V loop analysis and quantifying heart function metrics, specifically in terms of the heart workload; d) providing a breakdown of the effects of disease constituents on global heart function (eg, heart workload) to help predict the effects of interventions and plan the sequence of interventions in C3VD; e) quantifying other heart-function metrics, including LV end-diastolic pressure, instantaneous LV pressure, and contractility. None of the above metrics can be obtained non-invasively in patients, and when invasive cardiac catheterization is undertaken, the collected metrics cannot be as complete as what the novel method can provide. While such information is vitally needed for the effective use of advanced therapies to improve clinical outcomes and to guide interventions in patients, they are not currently accessible in clinical settings.
Figure 2. Diagnosis and monitoring in sample patient 1 from baseline to 90 days: this patient did not fully benefit form transcatheter aortic valve implantation (TAVI). Instead of improving the patient’s heart condition by reducing LV workload, TAVI caused an increase in LV workload. Example of workload breakdown analysis and prediction for effects of interventions in sample patient 2: right: P-V loops of the actual disease condition and prediction of several valve interventions. Left: predicted percent decrease in LV workload following valve interventions. Both mitral valve regurgitation (38% increase) and aortic valve stenosis and regurgitation (48% increase) substantially contributed to increasing the workload. This patient only underwent TAVI. However, considering this calculation, the decision to also perform mitral intervention at the time of aortic valve intervention should have been evaluated. AS, aortic stenosis; AR, aortic regurgitation; MR, mitral regurgitation; LV, left ventricle; LA, left atrium. We retrospectively received patients’ data from multicentre in which waiver of informed consent and data transfer were approved by their Institutional Review Boards.
CONCLUSION
The novel method, developed and verified by Keshavarz-Motamed,11,12 purposefully uses reliable and noninvasive input parameters measured by Doppler echocardiography to continuously calculate patient-specific hemodynamics to be used for diagnosis, monitoring, and prediction of cardiac function and circulatory status. This innovative method holds potential applications: a) in clinical trials, enabling the noninvasive analysis of cardiac and circulatory function metrics; b) as a diagnostic tool to noninvasively analyze cardiac function metrics for routine care, ambulatory care, or intensive and critical care units; c) as a patient monitoring tool, potentially integrated into personal wearable devices; and d) as a module incorporated into the software of Doppler echocardiography machines for enhanced diagnosis and prediction.
FUNDING
None.
CONFLICTS OF INTEREST
None.
REFERENCES
1. Savarese G, Lund LH. Global Public Health Burden of Heart Failure. Card Fail Rev. 2017;3:7-11.
2. Keshavarz-Motamed Z, Khodaei S, Rikhtegar Nezami F, et al. Mixed Valvular Disease Following Transcatheter Aortic Valve Replacement:Quantification and Systematic Differentiation Using Clinical Measurements and Image-Based Patient-Specific In Silico Modeling. J Am Heart Assoc. 2020;9:e015063.
3. Bastos MB, Burkhoff D, Maly J, et al. Invasive left ventricle pressure-volume analysis:overview and practical clinical implications. Eur Heart J. 2020;41:1286-1297.
4. Khodaei S, Abdelkhalek M, Maftoon N, Emadi A, Keshavarz-Motamed Z. Early Detection of Risk of Neo-Sinus Blood Stasis Post-Transcatheter Aortic Valve Replacement Using Personalized Hemodynamic Analysis. Struct Heart. 2023;7:100180.
5. Ben-Assa E, Brown J, Keshavarz-Motamed Z, et al. Ventricular stroke work and vascular impedance refine the characterization of patients with aortic stenosis. Sci Transl Med. 2019;11:eaaw0181.
6. Seppelt PC, De Rosa R, Mas-Peiro S, Zeiher AM, Vasa-Nicotera M. Early hemodynamic changes after transcatheter aortic valve implantation in patients with severe aortic stenosis measured by invasive pressure volume loop analysis. Cardiovasc Interv Ther. 2022;37:191-201.
7. Arnold SV. Calculating Risk for Poor Outcomes After Transcatheter Aortic Valve Replacement. J Clin Outcomes Manag. 2019;26:125-129.
8. Sarraf M, Burkhoff D, Brener MI. First-in-Man 4-Chamber Pressure-Volume Analysis During Transcatheter Aortic Valve Replacement for Bicuspid Aortic Valve Disease. JACC Case Rep. 2021;3:77-81.
9. Fischer-Rasokat U, Renker M, Liebetrau C, et al. Outcome of patients with heart failure after transcatheter aortic valve implantation. PLoS One. 2019;14:e0225473.
10. Khodaei S, Garber L, Abdelkhalek M, Maftoon N, Emadi A, Keshavarz-Motamed Z. Reducing Long-Term Mortality Post Transcatheter Aortic Valve Replacement Requires Systemic Differentiation of Patient-Specific Coronary Hemodynamics. Journal of the American Heart Association. 2023;12:e029310.
11. Keshavarz-Motamed Z. A diagnostic, monitoring, and predictive tool for patients with complex valvular, vascular and ventricular diseases. Sci Rep. 2020;10:1-19.
12. Keshavarz Motamed Z. Diagnostic, monitoring, and predictive tool for subjects with complex valvular, vascular and ventricular diseases. US &Canada patent US11844645B2, granted, 2023.
13. Bahadormanesh N, Tomka B, Kadem M, Khodaei S, Keshavarz-Motamed Z. An ultrasound-exclusive non-invasive computational diagnostic framework for personalized cardiology of aortic valve stenosis. Med Image Anal. 2023;87:102795.
14. Sadeghi R, Tomka B, Khodaei S, Garcia J, Ganame J, Keshavarz-Motamed Z. Reducing Morbidity and Mortality in Patients With Coarctation Requires Systematic Differentiation of Impacts of Mixed Valvular Disease on Coarctation Hemodynamics. J Am Heart Assoc.2022;11:e022664.
15. Sadeghi R, Tomka B, Khodaei S, et al. Impact of extra-anatomical bypass on coarctation fluid dynamics using patient-specific lumped parameter and Lattice Boltzmann modeling. Sci Rep. 2022;12:9718.
* Author for correspondence.
E-mail address: motamedz@mcmaster.ca (Z. Keshavarz-Motamed).
Percutaneous coronary interventions with drug-eluting stent (DES) implantation have become a well-established treatment for obstructive coronary artery disease, improving long-term outcomes.1 However, despite recent improvements including thinner strut platforms and more biocompatible polymers, the Achilles’ heel of DES strategy remains the risk of DES-related adverse events such as in-stent restenosis or stent thrombosis in the short term,2 along with an increase in hard clinical events at a rate of 2.0 to 3.5% yearly after the first year.3,4
Drug-coated balloons (DCB) have been developed as an alternative to percutaneous coronary intervention with DES implantation in selected populations for the treatment of coronary artery disease. The main advantage of this technology is its ability to deliver an antiproliferative drug to the treated lesion without leaving any layer of metal, which might cause late adverse events. Another advantage is the potential reduction in the duration or discontinuation of dual antiplatelet therapy, especially in patients at high risk of bleeding.
Several studies have investigated the role of DCB in real-world patients, who are those mainly affected by in-stent restenosis or de novo small vessel disease.5-9 The only randomized study of DCB in de novo small vessels with a clinical primary endpoint was BASKET-SMALL-2. This study demonstrated the noninferiority of DCB vs DES (vessel size 2-3 mm), which was maintained up to 3 years follow-up in terms of all clinical endpoints.5
The initial fear of leaving behind a residual coronary dissection, especially in de novo lesions, could limit the widespread use of DCB. However, it has been shown that a nonflow-limiting dissection after DCB treatment tends to heal during the first few months, with both the paclitaxel and sirolimus technologies, without leading to acute or subacute vessel closure.10,11
The main message regarding DCB is that they should be used as the final step of percutaneous coronary intervention and only when a proper lesion preparation has been performed with a fully expanded balloon of the correct size for the vessel, with accurate management of calcifications and no residual stenosis greater than 30% that could impair drug delivery to the vessel and limit the potential of this technology.
Recently, a new generation of DCB eluting sirolimus (SCB, Magic Touch, Concept Medical, United States) has been introduced that uses nanoparticles composed of a dual layer of phospholipids encapsulating the antiproliferative agent. Histopathologic studies have demonstrated therapeutic concentrations of the drug within the vessel wall for up to 60 days after percutaneous coronary intervention.12
Notably, the angiographic performance of this class of drug seems to be inferior to that provided by paclitaxel. The recently published TRANSFORM I trial showed that SeQuent Please DCB (B. Braun, Germany) outperformed SCB in terms of angiographic parameters at 6 months of follow-up, but without showing any difference in clinical endpoints. This lower performance of SCB seems to occur particularly in cases of complex lesions, emphasizing the importance of adequate lesion preparation, especially with the less lipophilic drug sirolimus (figure 1).13 Somewhat reassuringly, the performance of SCB in terms of clinical endpoints has been demonstrated in all-comer populations, especially in the prospective EASTBOURNE study, which showed a good safety and efficacy profile up to 2 years of follow-up in 2123 patients/2440 lesions.14
Figure 1. Differences in terms of types of lesion and outcomes among 2 top enroller centers for the TRANSFORM II trial. PCB, paclitaxel-coated balloon; SCB, sirolimus-coated balloon.
The next step to ensure wider use of this new generation DCB will be direct comparison with DES, as in the TRANSFORM II (NCT04893291) trial. This is an international, multicenter, prospective, investigator-driven, open-label, randomized (1:1) clinical trial designed to test the efficacy of SCB vs DES in native coronary artery vessels with diameters between 2.0 and 3.5 mm. Inclusion and randomization are being performed after adequate lesion preparation in the absence of flow-limiting dissection and acute vessel recoil. The study population has been calculated expecting the noninferiority of SCB in terms of target lesion failure at 12 months, and its sequential superiority in terms of net-adverse clinical events, including BARC 3-5 bleeding events. Interestingly, patients will be followed up clinically for 5 years to observe the potential superiority of DCB in the long-term. This trial, which includes 7 Spanish centers, is including patients at 40 centers allocated in 11 countries in Europe, Asia, and South America.15 By November 20th, 2023, 600 patients out of the planned 1820 had been enrolled.
The TRANSFORM II trial will be an essential test of the maturity of DCB in such an established, prognostically significant arena, challenging DES as the gold standard for the treatment of patients with native coronary artery disease.
FUNDING
None.
CONFLICTS OF INTEREST
B. Cortese serves on the advisory board or as a consultant for several companies producing or marketing DCB: Cordis, Medalliance, BBraun, Concept Medical, Medtronic, Innova HTS, and ANT.
REFERENCES
1. Neumann FJ, Sousa-Uva M, Ahlsson A, et al. 2018 ESC/EACTS Guidelines on myocardial revascularization. Eur Heart J. 2019;40:87-165.
2. Sarno G, Lagerqvist B, Frobert O, et al. Lower risk of stent thrombosis and restenosis with unrestricted use of 'new-generation'drug-eluting stents:a report from the nationwide Swedish Coronary Angiography and Angioplasty Registry (SCAAR). Eur Heart J. 2012;33:606-613.
3. Kufner S, Ernst M, Cassese S, et al. 10-Year Outcomes From a Randomized Trial of Polymer-Free Versus Durable Polymer Drug-Eluting Coronary Stents. J Am Coll Cardiol. 2020;76:146-158.
4. Brugaletta S, Gomez-Lara J, Ortega-Paz L, et al. 10-Year Follow-Up of Patients With Everolimus-Eluting Versus Bare-Metal Stents After ST-Segment Elevation Myocardial Infarction. J Am Coll Cardiol. 2021;77:1165-1178.
5. Jeger RV, Farah A, Ohlow MA, et al. Long-term efficacy and safety of drug-coated balloons versus drug-eluting stents for small coronary artery disease (BASKET-SMALL 2):3-year follow-up of a randomised, non-inferiority trial. Lancet. 2020;396:1504-1510.
6. Giacoppo D, Alfonso F, Xu B, et al. Paclitaxel-coated balloon angioplasty vs. drug-eluting stenting for the treatment of coronary in-stent restenosis:a comprehensive, collaborative, individual patient data meta-analysis of 10 randomized clinical trials (DAEDALUS study). Eur Heart J. 2020;41:3715-3728.
7. Wanha W, Bil J, Januszek R, et al. Long-Term Outcomes Following Drug-Eluting Balloons Versus Thin-Strut Drug-Eluting Stents for Treatment of In-Stent Restenosis (DEB-Dragon-Registry). Circ Cardiovasc Interv. 2021;14:e010868.
8. Cortese B, Di Palma G, Guimaraes MG, et al. Drug-Coated Balloon Versus Drug-Eluting Stent for Small Coronary Vessel Disease:PICCOLETO II Randomized Clinical Trial. JACC Cardiovasc Interv. 2020;13:2840-2849.
9. Cortese B, Testa G, Rivero F, Erriquez A, Alfonso F. Long-Term Outcome of Drug-Coated Balloon vs Drug-Eluting Stent for Small Coronary Vessels:PICCOLETO-II 3-Year Follow-Up. JACC Cardiovasc Interv. 2023;16:1054-1061.
10. Cortese B, Silva Orrego P, Agostoni P, et al. Effect of Drug-Coated Balloons in Native Coronary Artery Disease Left With a Dissection. JACC Cardiovasc Interv. 2015;8:2003-2009.
11. El Khoury A, Lazar L, Cortese B. The fate of coronary dissections left after sirolimus-coated balloon angioplasty:A prespecified subanalysis of the EASTBOURNE study. Catheter Cardiovasc Interv. 2023;102:979-986.
12. Cortese B, Kalkat H, Bathia G, Basavarajaiah S. The evolution and revolution of drug coated balloons in coronary angioplasty:An up-to-date review of literature data. Catheter Cardiovasc Interv. 2023;102:1069-1077.
13. Ono M, Kawashima H, Hara H, et al. A Prospective Multicenter Randomized Trial to Assess the Effectiveness of the MagicTouch Sirolimus-Coated Balloon in Small Vessels:Rationale and Design of the TRANSFORM I Trial. Cardiovasc Revasc Med. 2021;25:29-35.
14. Cortese B, Testa L, Heang TM, et al. Sirolimus-Coated Balloon in an All-Comer Population of Coronary Artery Disease Patients:The EASTBOURNE Prospective Registry. JACC Cardiovasc Interv. 2023;16:1794-1803.
15. Greco A, Sciahbasi A, Abizaid A, et al. Sirolimus-coated balloon versus everolimus-eluting stent in de novo coronary artery disease:Rationale and design of the TRANSFORM II randomized clinical trial. Catheter Cardiovasc Interv. 2022;100:544-552.
Simply stated, the goal of diagnostic coronary angiography is to distinguish the cause of a patient’s chest pain from 1 of 4 endotypes: a) epicardial stenosis; b) coronary spasm; c) coronary microvascular disease (CMD); and d) —equally important—noncoronary chest pain. Crucially, the latter is a diagnosis of exclusion and consequently cannot be confirmed without formal assessment of the other mechanisms (figure 1). Despite this truism, the interpretation of most coronary angiograms is limited to simple “eyeballing” of an epicardial “shadowgram”. This approach has a low diagnostic yield with 40% of patients found to have no significant epicardial stenoses—an entity known as angina with no obstructive coronary arteries (ANOCA).1 Despite the presence of typical angina or evidence of ischemia during noninvasive testing, these patients, are frequently nonchalantly dismissed without a formal diagnosis.
Figure 1. Patients with compelling, recurring, and debilitating chest pain should undergo catheterization with coronary angiography and—when needed—coronary function testing to unravel the mechanism of their pain. Noncoronary chest pain is a diagnosis of exclusion and consequently can only be confirmed if the 3 other mechanisms have been assessed. FFR, fractional flow reserve; PPG, pullback pressure gradient.
This very large group of patients is heterogeneous, and establishing the underlying cause of ANOCA requires a thorough coronary function testing (CFT) protocol that includes diagnostic angiography, provocation testing for microvascular or epicardial vasospasm, and assessment of CMD.2 In many centers, however, diagnostic angiography is rarely complemented with CFT. Among those that do, testing is often incomplete, with the result that patients often do not receive a diagnosis of the underlying cause of their ANOCA or benefit from potential endotype-specific treatments. Possible explanations for this behavior include a lack of familiarity with the causes of ANOCA, a lack of knowledge of available testing modalities, concerns about the accuracy of tests, and a belief that the underlying diseases are untreatable.
In a recent article published in REC: Interventional Cardiology, Rinaldi et al.3 describe their single-center experience of the implementation of a specific ANOCA diagnostic and therapeutic protocol at Hospital Clínic in Barcelona, Spain. In this program, all patients with ANOCA underwent systematic CFT including bolus thermodilution for the calculation of coronary flow reserve and the index of microvascular resistance, as well as intracoronary provocation testing to assess epicardial or microvascular spasm. Based on the results of these tests, patients were classified into 4 endotypes: a) microvascular angina (MVA) (CMD or microvascular spasm); b) vasospastic angina (epicardial spasm); c) both MVA and vasospastic angina; and d) noncoronary chest pain.
The authors demonstrated that, as a result of the identification of specific ANOCA endotypes, there were significant increases in targeted medical prescriptions such as beta-blockers, nondihydropyridine calcium channel blockers, and long-acting nitrates. While this did not translate into a statistically significant improvement in quality of life between baseline and 3 months, angina significantly improved in terms of physical limitation, angina stability, and disease perception. Importantly, the protocol was shown to be safe, with only 3 minor adverse events being reported, all occurring during acetylcholine administration (transient bradycardia, paroxysmal atrial fibrillation with spontaneous cardioversion).
This work has significant strengths that should be highlighted. The CorMicA trial provided the first evidence from a randomized controlled trial of the benefits of systematic CFT with targeted medical therapy.4 However, to date, scarce real-world data have been available on the implementation of such protocols in routine clinical practice. As such, this small, real-world, observational study is strongly welcomed. More than the clinical results obtained in a relatively small number of patients, the work by Rinaldi et al.3 is particularly worthwhile for several methodological aspects, 3 of which are discussed below.
(i) Which patients should enter such a program and how? Patients were screened at a specific outpatient clinic and their inclusion was based on well-standardized criteria. Ideally, only patients with compelling, recurrent and invalidating symptoms should undergo CFT. The usefulness of such a program is significantly reduced by the referral of patients with unconvincing symptoms, or those with a high pretest probability of epicardial disease. Notably, the increasing role of coronary computed tomography angiography for the screening of epicardial disease in patients with angina currently allows diagnosis of ANOCA without the use of invasive diagnostic angiography and patients can thus be referred directly for CFT.
(ii) How should CFT be performed? As described by the authors, both microvascular function and coronary vasomotion should be investigated in a strictly standardized manner, preferably in the left anterior descending artery. However, the order of these tests is debatable. In our opinion, it does not make sense to investigate endothelial function and coronary vasomotion with a guidewire in the coronary artery or when the patient has already received vasoactive medications such as nitrates and calcium channel blockers. Consequently, we believe that acetylcholine testing should come first, with epicardial vasodilation being induced with nitrates at the end of acetylcholine testing. The latter also represents an important step (and good clinical practice) before commencing wire-based measurements of microcirculatory function. As for the choice of testing modality, bolus thermodilution should be replaced by continuous thermodilution as it enables the calculation of coronary flow reserve, absolute microvascular resistance (Rµ) and microvascular resistance reserve from absolute volumetric flow rather than from surrogates of flow.5 Future studies are expected to clarify the clinically relevant cutoff values for the indices derived from continuous thermodilution. These values should allow a more robust definition of CMD, which is currently an unmet need.
(iii) How should these patients be followed up? Patients should be followed up by the same physicians in a dedicated outpatient clinic, and be asked about their symptoms in a structured and systematic way. In this regard, the authors should be commended for the use of structured questionnaires to assess angina and quality of life. The classic Canadian Cardiovascular Society grading system for angina is not appropriate for ANOCA patients as their symptoms often differ from those reported by patients with epicardial disease. Moreover, objective signs of ischemia are often lacking. In the future, it is likely that patients will be given an app on their smartphone to closely monitor their symptoms.6
Overall, as with any new program, it is important to recognise that there is a learning phase. However, with a well-structured and standardized program, such as that proposed by Rinaldi et al.,3 this learning phase is likely to be short. There is now a need for future work addressing the implications of such a protocol on both time and cost in routine clinical practice. For example, in the setting of a busy, real-world catheterization laboratory, how much time, on average, does such a protocol add to the length of the procedure? Furthermore, what are the cost implications, and are they sufficiently counterbalanced by an increase in quality of life and/or symptom control?
To conclude, there are now strong clinical grounds for the systematic implementation of CFT in ANOCA patients. Furthermore, as demonstrated by Rinaldi et al., a standardized and robust testing program can be effectively implemented in real-world practice.3 Validation of clear diagnostic criteria for CMD is now needed for the results of CFT to be easily interpreted and acted upon. Patients and their referring clinicians deserve nothing less.
FUNDING
No funding was received to assist with the preparation of this editorial.
CONFLICTS OF INTEREST
T. Mahendiran is supported by a grant from the Swiss National Science Foundation (SNSF). B. De Bruyne has a consulting relationship with Boston Scientific, Abbott Vascular, CathWorks, Siemens, and Coroventis Research; receives research grants from Abbott Vascular, Coroventis Research, Cathworks, Boston Scientific; and holds minor equities in Philips-Volcano, Siemens, GE Healthcare, Edwards Life Sciences, HeartFlow, Opsens, Sanofi, and Celyad.
REFERENCES
1. Patel MR, Peterson ED, Dai D, et al. Low Diagnostic Yield of Elective Coronary Angiography. N Engl J Med. 2010;362:886-895.
2. Jansen TPJ, Konst RE, Elias-Smale SE, et al. Assessing Microvascular Dysfunction in Angina With Unobstructed Coronary Arteries. J Am Coll Cardiol. 2021;78:1471-1479.
3. Rinaldi R, Spione F, Verardi FM, et al. Angina or ischemia with no obstructed coronary arteries:a specific diagnostic and therapeutic protocol. REC Interv Cardiol. 2023. https://doi.org/10.24875/RECICE.M23000418.
4. Ford TJ, Stanley B, Good R, et al. Stratified Medical Therapy Using Invasive Coronary Function Testing in Angina:The CorMicA Trial. J Am Coll Cardiol. 2018;72:2841-2855.
5. De Bruyne B, Pijls NHJ, Gallinoro E, et al. Microvascular Resistance Reserve for Assessment of Coronary Microvascular Function:JACC Technology Corner. J Am Coll Cardiol. 2021;78:1541-1549.
6. Nowbar AN, Howard JP, Shun-Shin MJ, et al. Daily angina documentation versus subsequent recall:development of a symptom smartphone app. Eur Heart J Digit Health. 2022;3:276-283.
* Corresponding author.
E-mail address: Bernard.de.bruyne@olvz-aalst.be (B. De Bruyne).
- Percutaneous pulmonary valve implantation in native outflow tracts: has the time come?
- Transcatheter aortic valve replacement for noncalcified aortic regurgitation. Where are we now?
- Vascular closure devices: the jury is still out
- Optimizing radiation exposure in interventional cardiology: are current doses appropriate?
Subcategories
Editorials
Original articles
Editorials
Infective endocarditis in surgical versus transcatheter aortic valve implantation. Same incidence and same prognosis?
aServicio de Cardiología, Hospital Clínico Universitario de Valladolid, Instituto de Ciencias del Corazón (ICICOR), Valladolid, Spain
bCentro de Investigación Biomédica en Red de Enfermedades Cardiovasculares (CIBERCV), Spain
Original articles
Debate
Debate: Intravascular imaging in percutaneous revascularization procedures
For a more widespread approach
aServicio de Cardiología, Consorcio Hospital General Universitario de Valencia, Valencia, Spain
bServicio de Cardiología, Hospital QuironSalud Valencia, Valencia, Spain
For an optimized and still selective approach
aServicio de Cardiología, Hospital del Mar, Barcelona, Spain
bCentro de Investigación Biomédica en Red de Enfermedades Cardiovasculares (CIBERCV), Spain